Definition
Nuclear Magnetic Resonance Spectroscopy, or “NMR,” is a process used to find out information about a compound’s magnetic properties. It does so by recording the magnetic spectral patterns given off by the nuclei within a sample’s atoms. Using NMR, researchers can determine the molecular structure of a compound.
Overview
Scientists use a machine called an NMR Spectrometer, which processes using electromagnetic radiation, such as radio waves. An NMR Spectrometer pulses a radio wave at the sample, which excites the atoms within the sample. At specific frequencies, the atoms will resonate, and return a signal. These signals are specific to certain kinds of atoms and change depending on how the atoms are bonded. The returning frequencies detected by the sensitive machine are therefore specific to each molecule. This information can be used to determine the size and shape of the original molecule.
Each singular atom gives off enough of a resonance shift to be traced by NMR machinery, and this is greatly dependent on and affected by the effective magnetic field at the nucleus. This resonance changes as an atom forms more connections with other atoms. We can draw upon these resonance patterns to begin to understand the details of a molecule’s three-dimensional structure and the functional groups that form it.
Advantages of NMR Spectroscopy
Years upon years of research in chemistry has helped scientists create the immense spectral library that we now use as a reference tool for taking some of the mystery out of identifying unknown substances. We now know which spectral patterns to expect from which functional groups and can infer structural, chemical, and magnetic information from an NMR reading. It is thus no overstatement to say that NMR has helped us develop a deeper understanding of the world around us.
Compared with mass spectrometry, NMR spectroscopy contains several advantages. First of all, there is no need to purify or derive a substance before analysis. NMR is able to measure and observe all of the atoms within the sample. Second, NMR is a quantitative measurement and therefore does not need as much analysis as mass spectrometry to decipher the results. However, NMS may not be the appropriate tool for some applications.
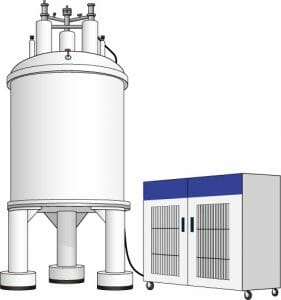
NMR and Unknown Compounds
The resonance readings we obtain from NMR spectroscopy lends us the ability to decipher an unknown compound’s molecular structure and its “purity.” Purity is defined as a substance which contains only one kind of molecule. Sometimes, solvents or other chemicals may stay within a sample and decrease its purity. If we were testing a mystery substance, then, we would compare the spikes in our NMR readings to the vast spectral libraries in existence and then make inferences about the compounds basic structure.
Measuring Each Atom
Our readings let us infer a couple of things. First, the length of a spike in our NMR readings will reflect the relative proportion of said atom in our sample’s skeleton. Therefore, we can estimate how many hydrogen atoms or methane groups are in our unknown compound based on the relative length of the spike compared to those given off by the other atoms.
Understanding the Position of Atoms
Second, NMR spectroscopy also gives us information about the relative position of our atoms. A hydrogen atom can give off several different resonance signals depending on its neighboring atoms or groups. For example, a hydrogen atom located next to a polar group, such as an oxygen-containing carboxyl group, will give off a higher NMR reading than a hydrogen atom neighbored by non-polar methane groups.
A hydrogen atom bonded to a polar atom, on the other hand, will have an even higher NMR reading due to its increased resonance shift. In general, the pattern we find is that polar relationships will give off high NMR readings while non-polar accomplish the opposite. NMR works spectacularly well for functional groups. Hydroxyl groups, amine groups, carboxyl groups, and more have characteristic NMR resonance shifts that are an automatic tell. When we find them, we can really begin to visualize our molecule.
Once our basic structure is understood, our NMR results can help us infer several things about the compound’s molecular conformation and its physical properties (i.e. boiling, melting points, phase changes) based on the molecular components present and their polarity.
The Principles of NMR
The guiding principle behind NMR lies in the fact that nuclei have two special properties: they can spin and they are charged. These properties cause nuclei to react to a magnetic field. If we were to run an electric current or apply an external magnetic field, this would allow an upward energy transfer to a higher state. This energy transfer is reflected at a certain wavelength and radio frequency.
Once the spin returns to its baseline, the emitted energy from the drop will be read by the NMR machinery. Likewise, the application of an external magnetic field can cause a nucleus to either spin in the same direction, or against the direction, of the magnetic field. A nucleus with the opposite spin will have higher energy. A nucleus spinning in the same direction will have lower energy.
Furthermore, the resonant frequency as it applies to NMR spectroscopy will be affected by the electron shielding. The general rule is that the more electronegative the nucleus is, the higher the resonant frequency expected. Further, the more electronegative, or “electron withdrawing” a group is the lower the chemical shift. On the other hand, the most “electron donating” groups will have the highest chemical shifts. The rise in chemical shift can be due to many factors, including the delocalization of current that occurs in aromatic groups that can distribute current across the groups.
NMR Patterns and Signal Splitting
While we’ve briefly discussed a few things to look for when interpreting NMR readings, it’s important to discuss chemical splitting in more detail and summarize a few points.
Interpreting NMR Signals:
- The number of signals will reflect the number of equivalent (“like”) protons
- The intensity or size of the signal will infer a ratio of that specific type of proton
- The position of the signal will infer “chemical shift,” where the position of the peak in the NMR spectrum will indicate how de-shielded or shielded the proton is
- Signal Splitting will be represented by the number of peaks/lines that a proton signal will split into depending on the other proton neighbors.
Signal Splitting
The process of signal splitting is an important concept in NMR and gives us vital information about the protons in our molecule. This signal splitting phenomenon is one where we see a proton signal will “split” into several smaller peaks all dependent on their hydrogen neighbors.
When there are no adjacent hydrogens, we will observe a single peak. However, when one hydrogen is adjacent to another hydrogen, the resonance will split in two, called a doublet. Further, when two hydrogens are found on adjacent atoms, we will see three peaks called a triplet signal. On the other hand, when there are three hydrogens on the adjacent atoms we will see the resonance split to four peaks called a quartet.
The NMR resonance will be predictably split into N + 1 peaks, where N represents the number of hydrogens on the adjacent functional groups.
Quiz