Definition
Semantic memory is a cognitive sub-topic in psychology regarding the human ability to remember knowledge and facts. Though studied for decades, much about it is still unknown, such as the specific brain parts used in its processing. While there is some overlap with episodic memory’s temporal and frontal brain structures, it is speculated that the major structures for semantic memory is found in the inferolateral temporal lobe. Studies are ongoing to determine how exactly semantic memories are stored and retrieved. However, several effects- including category size, typicality, false-relatedness, context, familiarity, and fast- true- have been postulated. Though age is known to affect memory, semantic memory has not been found to diminish naturally with age. However, those with specific diseases- such as Alzheimer’s, semantic dementia, and herpes simplex encephalitis- may have temporal lobe degradation that can affect semantic memory.
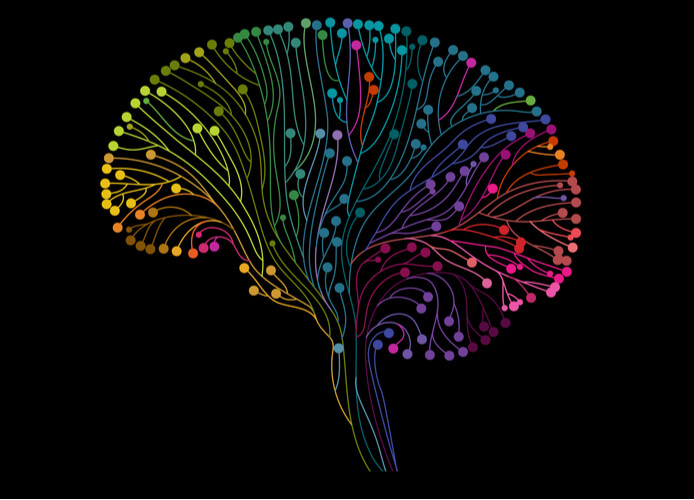
Background
Neurons and the Brain
Semantic memory is a cognitive ability possible due to neural connections in the brain. Neurons (or nerve cells) are individual cells that make up the entirety of the nervous system. They are able to quickly stimulate adjacent neurons and spread throughout the body almost instantly by use of electrical signals. This is done as one neuron synapses on the next, where the electrical signal turns chemical before becoming electrical again at the adjacent neuron. Neurons make up all aspects of the nervous system, including the central nervous system (which is the brain and spinal cord) and peripheral nervous system (which are all other neurons).
Several structures in the brain have been studied in relation to semantic memory. Generally, the brain is broken down into four main sections: the cerebrum (also called the cerebral cortex, or just cortex), cerebellum, diencephalon, and brain stem. The cerebrum is further broken down into lobes, including the frontal, parietal, temporal, and occipital lobes. While certain regions and lobes may be associated with specific functions, many functions require multiple brain structures and regions.
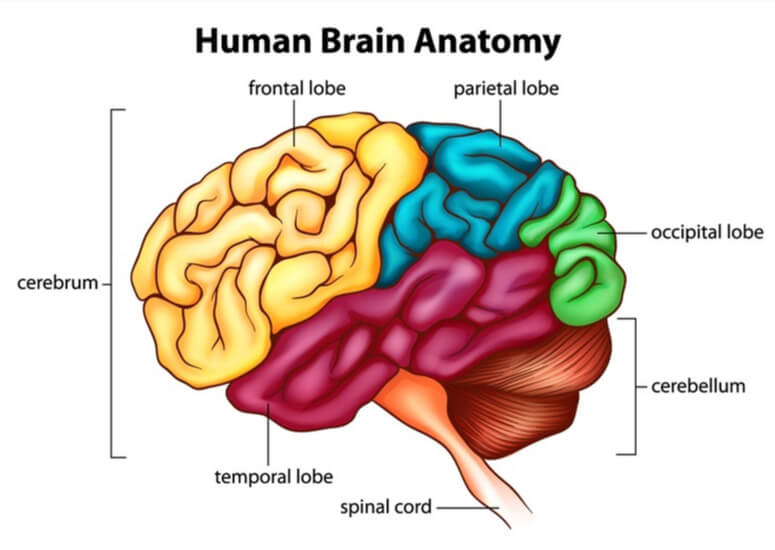
As will be discussed later on in this article, semantic memory is speculated to use multiple structures throughout the brain. Primarily, the structures hypothesized to be important are found in the cerebrum, specifically at the temporal lobe. However, studies continue to look at alternative regions and less prominent lobes to better determine the full capabilities of semantic memory.
Memory
Memory is defined as the retention of learned information. It is possible because neurons have plasticity, where neurons remember and make new connections throughout the brain to send and receive information. There are two forms of memory: short- term and long- term. Short- term memory (also known as working memory) only lasts for a small time period to allow people to complete tasks.
Long- term memory undergoes more intense processing in order to be stored in the brain for longer time periods. It is broken down into declarative vs non-declarative memory (also known as explicit vs. implicit memory). Non-declarative memory includes skills, habits, classical conditionings, and non- associative learning. Specifically, this form of “memory” does not actually require access to stored memory content. It is instead formed by non-conscious abilities that are capable of altering behavior. Meanwhile, declarative memory is the conscious access to facts and events. It is broken down into semantic and episodic memory, where semantic memory is the conscious access to facts and episodic memory is the conscious access to events.
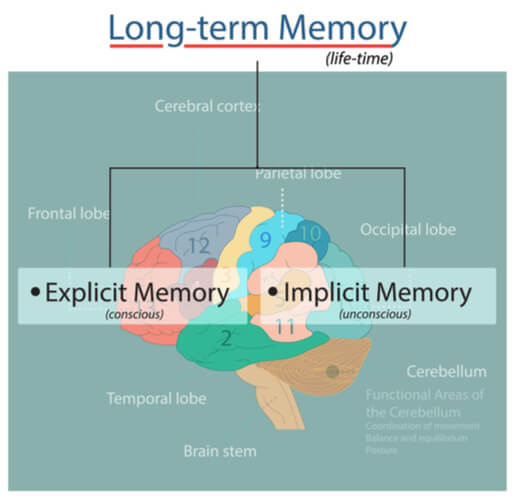
Memory processing is broken down into four stages: encoding, storage, consolidation, and retrieval. Encoding is information intake by the senses, while storage is the stable record of the information in our brains. Meanwhile, consolidation is the slow process in which memory transitions from short- term to long- term through repeated connections. Lastly, retrieval is the access to the stored information by neural connections. Generally, the structures involved in memory processing includes temporal structures (such as the hippocampus, parahippocampus, and amygdala), diencephalon structures (including the thalamus and mammillary bodies), the prefrontal cortex, and the cerebellum.
Semantic Memory
Semantic memory is a sub-topic in psychology regarding the ability to remember knowledge and facts. These facts are impersonal and can thus be applied across multiple scenarios. Examples include knowing how many feet are in a mile, what colors make up the rainbow, and even the vocabulary to complete a crossword puzzle. It is a form of declarative memory, as is its counterpart- episodic memory. However, episodic memory– defined as the human potential for recalling past experiences- is differentiated from semantic memory due to its need to be personal. Specifically, semantic memory is learned information that is stored into memory. There is some crossover between the two however, as personal experiences can help solidify learned information. In fact, research indicates that learning from mistakes is more likely to be due to episodic recollection than the semantic learning process itself.
While semantic memory has been studied for several decades, much about it is still unknown. However, multiple studies have tried to determine its process. Methodology ranges in quantitative and qualitative measurements, causing varying results that lead to multiple potential explanations for semantic memory. Multiple “effects” have been created as a result. As will be described later in this article, some semantic memory effects include category size, typicality, false-relatedness, context, familiarity, and fast- true.
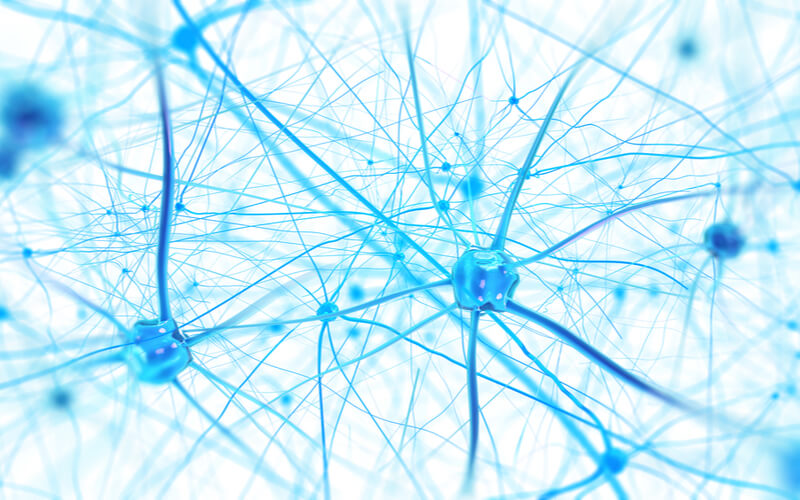
Since semantic memories contain factual information for both specific details and broad concepts, a conceptual system is needed for organization. This leads into categories and instances. Categories are broad groups split into supersets and subsets, while instances are specific examples. As a common example, “robin” is an instance, while “bird” is the category. “Bird” is then a subset of another category- “animals”- and so on.
Instances have both defining features and characteristic features. Defining features are essential to the existence of the category, while characteristic features are common but not required. (Example. Defining feature: Birds have features. Characteristic feature: Birds can fly.) The mind organizes categories from most definitive to most characteristic.
Associated Structures in Semantic Memory
In episodic memory, the medial temporal structures are mostly used for memory storage, while the frontal structures are for recollection and action. These structures include the hippocampus, parahippocampus, and the prefrontal cortex. While there is some overlap between the functions and potential structures for episodic memory and semantic memory, the exact structures for semantic memory are not entirely clear. Research has indicated that the inferolateral cortex may have a large role in storage and recollection, given its degradation in diseases decreasing semantic memory.
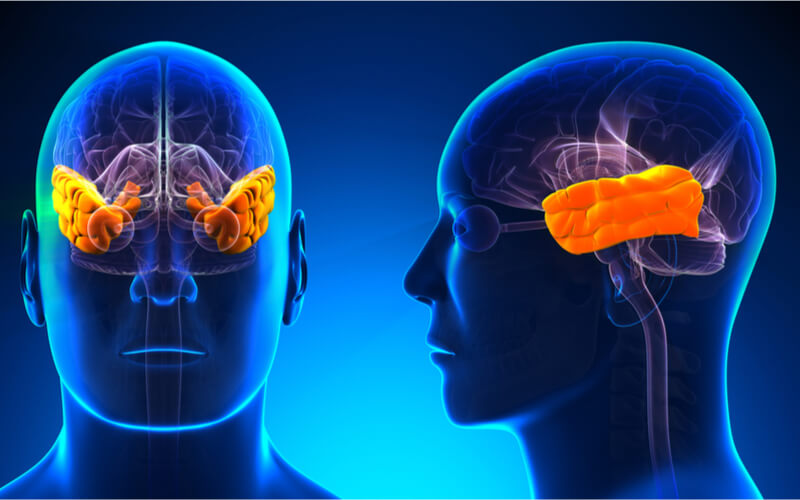
Currently, the thalamus and occipital lobes are speculated to be important for semantic memory, given their functions in sensory intake. It is then hypothesized that the occipital cortex begins semantic memory processing before reaching the inferolateral temporal lobe. Research also shows that the hippocampus, neocortex, amygdala, cerebellum, and basal ganglia may all play roles in semantic memory. However, the left inferior frontal cortex was specifically found to be important for word retrieval, and the fusiform gyrus (of the temporal lobe) was important for reading and defining words.
Semantic Memory Effects
Category Size Effect
The category size effect was one of the first and most important findings in relation to semantic memory. Category size is the idea that individuals are able to verify smaller categories quicker than larger categories. For example, the category “bird” is smaller than the category “animal”. Therefore, people tend to identify a “robin” as a “bird” faster than they would recognize a “robin” as an “animal”. Alternatively, reverse category size effects can also be possible, where some individuals may be able to recognize larger categories faster than small categories.
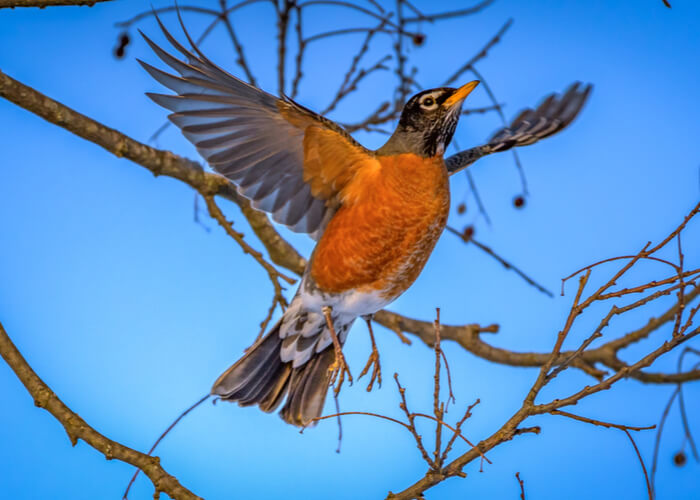
This discrepancy may be due to the methodology in which category size is measured. The most common method used is nested triplets, which are “instance”-“subset”-“superset” groups (example: “robin”-“bird”-“animal”). This grouping allows individuals to follow a stepwise pattern where the smaller category logistically fits into the larger category. In other words, the “instance” has features that fit “subset”, which has features that fit the “superset”.  (Example: A “robin” is a type of “bird” is a type of “animal”.) This is much easier for the mind to process as compared to the opposite. (Example: “Animals” include “birds” include “robins”.)
While category size is one of the more productive effects studied, false triples can also be created. False triples can incorrectly lead to instances being placed into larger categories, skewing the overall measurements of the study and negatively affecting results. Alternatively, individuals can estimate the absolute size of specific categories by counting the number of varying instances produced within a specific time period. This methodology can counterintuitively lead to some categories appearing larger than others, thus minimizing the effect.
Typicality Effect
The typically effect (also referred to as the relatedness effect)Â is the idea that the mind registers specific examples of a category faster when the example is considered to be more common, or “typical”. For example, consider the category “bird”. The instance “robin” is considered to be more representative of “birds” compared to “chicken”. This may be because “chicken” is more commonly considered a “farm animal” or even “food”. Therefore, the mind verifies “a robin is a bird” faster than “a chicken is a bird”.
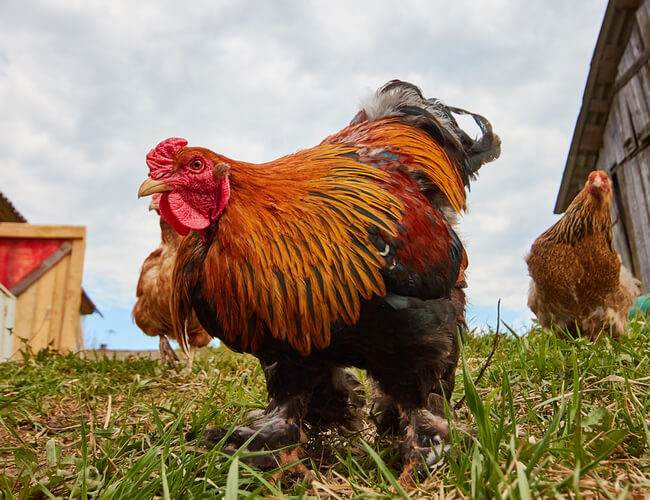
These types of verifications can be measured in two different ways: through production frequency (where the relative frequency to produce a concept from a stimulus is measured) and rating tasks with terminology such as “similar”, “related”, “associated”, and so on.
Typicality is asymmetrical, where “instance”- “category” order plays a role in how related the two may be perceived. For example, “insect” is commonly thought of following “butterfly”, but “butterfly” is not as commonly thought of following “insect”. Typical members of a category usually have several shared features. As a result, these members tend to be the main references for the category in the mind, and they are usually learned during childhood. When looking at order importance, production frequency methodology is needed, as rating systems have not been found to produce significant results for this parameter.
False- Relatedness Effect
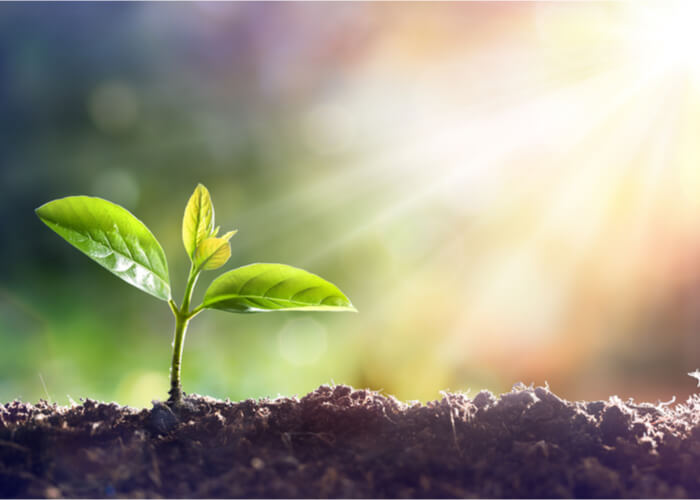
The false-relatedness effect (somewhat similar to the typicality effect) describes an individual’s speed in verifying instances and categories that appear to be related. In other words, it takes longer for individuals to reject incorrect “instance”-“category” pairs when the instance is in a similar category to the one listed. This is compared to individuals rejecting incorrect “instance”- “category” pairs when the instance is listed with a noticeably different category. For example, the instance “tree” falls into the category “plant”. However, it takes longer for individuals to reject “tree” as an “animal” compared to rejecting “brick” as an “animal”. Because “plants” and “animals” have some similar features as subcategories of “life”, it is thus easier to reject an instance that is not living.
Context Effect
The context effect (also similar to the typicality effect) compares pairs and groups when listed together. This is the idea that characteristics found in an instance, pair, or group affect how fast individuals react to other instances, pairs, and groups in the list. Experiments on the context effect found that it takes longer for the mind to process both true pairs and unrelated incorrect pairs when listed together with related incorrect pairs. This concept was consistent when comparing atypical true pairs vs incorrect pairs. Additionally, it was found that when part of the list changed, reaction time to the rest of the list also changed. Specifically, when related but incorrect pairs were replaced with unrelated incorrect pairs, the reaction time to process true pairs also increased.
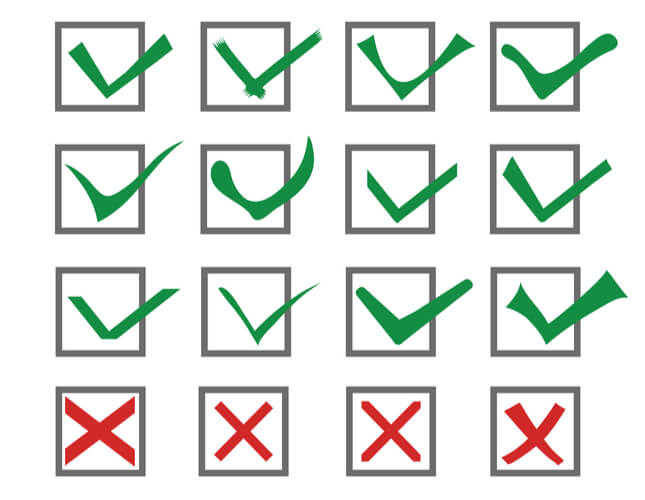
The context effect is also important when making “some”-“all” comparisons. Originally, it was thought that “some” statements were processed faster in the mind. However, it was later found that incorrect “all” statements tend to contain more related pairs compared to the “some” statements, which tended to be more opposing. Therefore, “some” statement processing slightly increased reaction time, where the examples used can affect the ease to distinguish right and wrong.
Familiarity Effect
The familiarity effect is the idea that familiar instances increase reaction time better than the instance itself. This idea came from a study that found increased familiarity led to decreased reaction time. This then produced the idea that the time required to understand an instance was not “preset”. Instead, time processing was better represented by familiarity when compared to any other effect.
It can be argued that characteristic comparisons from this study may have affected reaction time aside from instance familiarity. An additional study compared “instance”- “subset”- “superset” triples, where the instance matched the subset better in one group and the superset better in the other group. Category size had a larger effect in the first group (similar to the “robin”-“bird”-“animal” example), while typicality effect had a larger effect in the second group. This discrepancy could be due to the “instance” and “subset category” being more unrelated (as this group had the instance better matching the superset category), and thus less likely to follow category size.
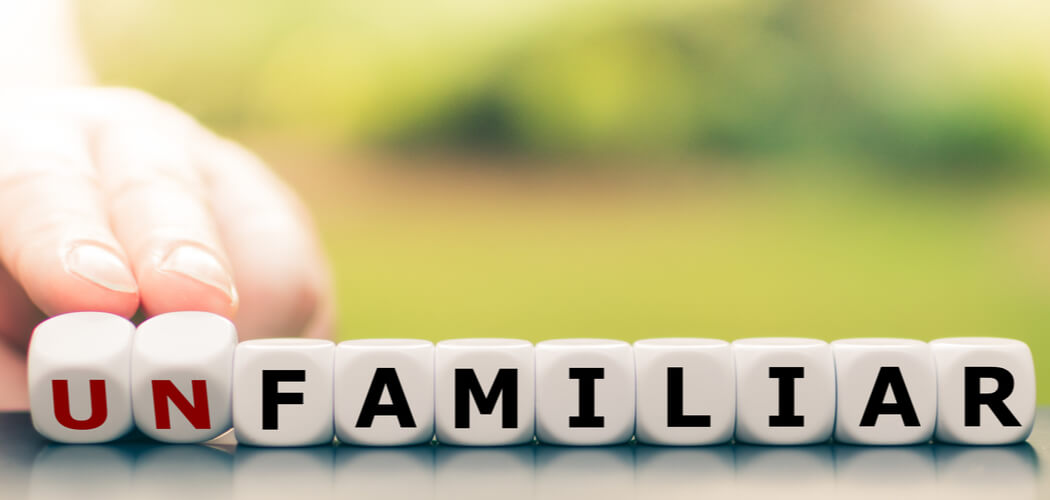
Connecting this study to the familiarity effect, it was speculated that the smaller subsets of the second group in this study were less familiar, thus leading to increased reaction times. In an additional study, extended time was given to individuals in order to study subsets before seeing the instance. Following this, reaction time decreased when the instance was shown. This may be due to the individuals being able to familiarize themselves with the subsets given.
Fast- True Effect
The fast- true effect has not been as heavily studied compared to the effects previously mentioned, but it may also play a large role in semantic memory. This effect initially found that “true” pairs had faster reaction times compared to “false” pairs in most studies completed. Alternatively, a few studies did not find a difference, while one found that “false” pairs were processed faster. It was also found that the fastest “true” times were also quicker than the fastest “false” times.
Semantic Memory Disorders
While it is commonly regarded that memory typically declines with age, research has shown that only specific memory types may decline. This includes episodic memory, where older individuals may find it harder to remember personal events and make new ones. However, evidence has shown that semantic memory is not typically affected by age. In fact, it may even slightly improve with age. In studies comparing older vs younger individuals’ ability to respond to vocabulary and fluency tests, semantic memory appeared to be fairly stable. Studies that did find decreased semantic memory however (such as a decreased ability to name common objects and determine words from their definitions), led to speculations that the memories themselves are not damaged, but the retrieval processes may be. This is further supported by these individuals having slight speech impairments and being more likely to have spelling errors.
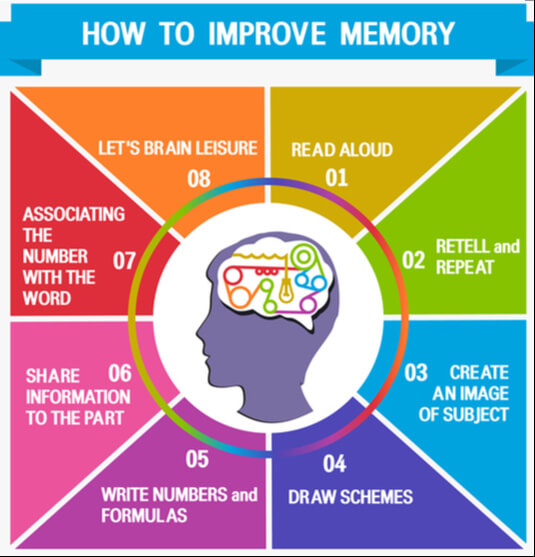
While semantic memory is largely well maintained, disorders can still result in some affected semantic memories. Cognitive tests are usually required to monitor information input and output to determine how memory may be impaired. These tests can include category fluency (listing instances in specific category), confrontation naming (naming what’s in a picture), naming to description (naming the word to fit a definition), verifying semantic attributes (confirming if specific features fit an instance given), amongst others.
Alzheimer’s Disease
Alzheimer’s Disease is the most common neurodegenerative disease, with symptoms ranging from memory loss to dysfunction in decision-making to decreased visuospatial and language capabilities. Scientists are still unsure of how exactly patients come to have the disease. However, it is known that the buildup of amyloid-beta (Aβ) plays a key role in its pathophysiology.
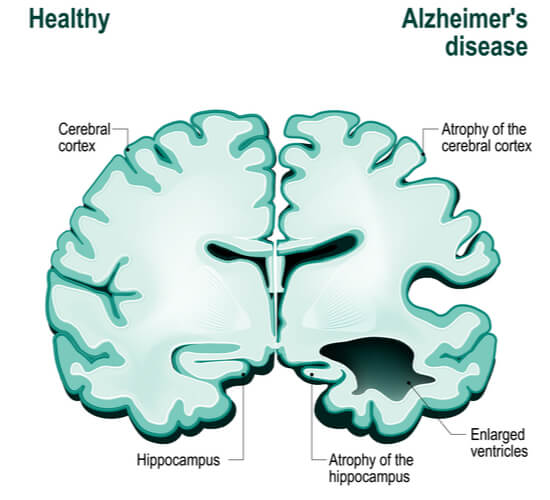
Generally, episodic memory is impaired in patients with Alzheimer’s disease, while semantic memory may not be affected at all. However, it is important to note that semantic memory impairment can occur, which can be early on in disease development. While episodic memory loss is a direct result in hippocampal damage, it is speculated that any semantic memory loss may be due to disease dispersal into the temporal neocortex proper. It is unknown if semantic memory decreases because of lost information or lost information retrieval. However, studies indicate that lost retrieval is more likely. This semantic memory disorganization is shown through improper ordering of information and incorrectly naming pictured items.
Semantic Dementia
Semantic dementia is the deterioration of semantic memories in a way that affects factual knowledge, instance recognition, and language processes. Specifically, detailed facts are more likely to be lost compared to general facts. (Example: The patient will remember that an elephant is an animal but does not remember that elephants have long trunks.) Patients with semantic dementia typically have intact episodic memory, but struggle to remember words and familiar instances. Despite this, visuospatial information, the frontal “decision making” structures, and non-verbal problem- solving skills are not impaired. Anatomically, there is degradation at the inferolateral temporal lobe. Studies continue to determine what other structures can be damaged and lead to impaired semantic memory, in addition to the following disease: herpes simplex encephalitis.
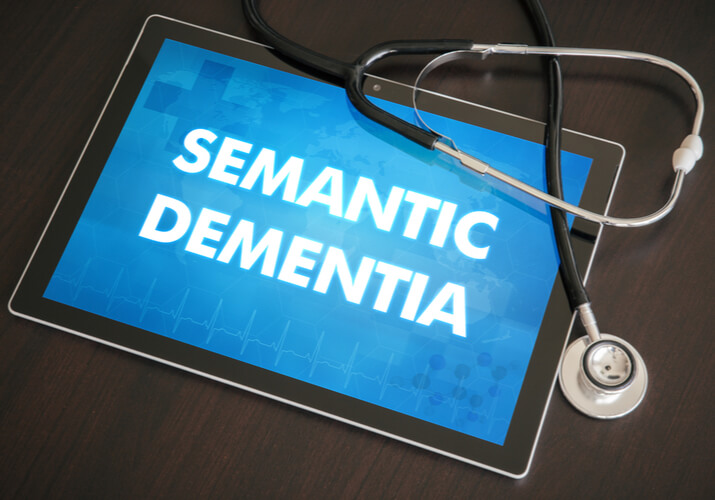
Herpes Simplex Encephalitis
Herpes simplex virus encephalitis is a rare disease in which the herpes simplex virus infects the central nervous system. This can be fatal if not treated quickly. Typically, the virus attacks the frontal and temporal lobes, which can affect multiple brain functions. This can include personality and cognitive alterations, as well as induced aphasia (language and communication disorders) and seizures. As a result of this disease, the inferolateral temporal lobe can be attacked, and thus semantic memory can be affected.
Notably, individuals with herpes simplex encephalitis are more likely to struggle remembering living things compared to manmade objects. From studying these individuals, research indicates that functionalism is separated in the brain. Also, that representations of different category types are located in different regions. As an additional hypothesis, living things may have more “perceptual” differences, where manmade things have more “functional” differences. This indicates that it may be easier for the mind to recall functions better than perceptions.
Quiz