Definition
An action potential is the result of a very rapid rise and fall in voltage across a cellular membrane, with every action potential (impulse) similar in size. The response of a nerve or muscle cell to an action potential can vary according to how frequently and for what duration the action potentials are fired. An action potential requires an influx of positive ions to produce a specific change in voltage (threshold value). It occurs after a certain degree of internal cell membrane depolarization – a positive increase in electrical charge.
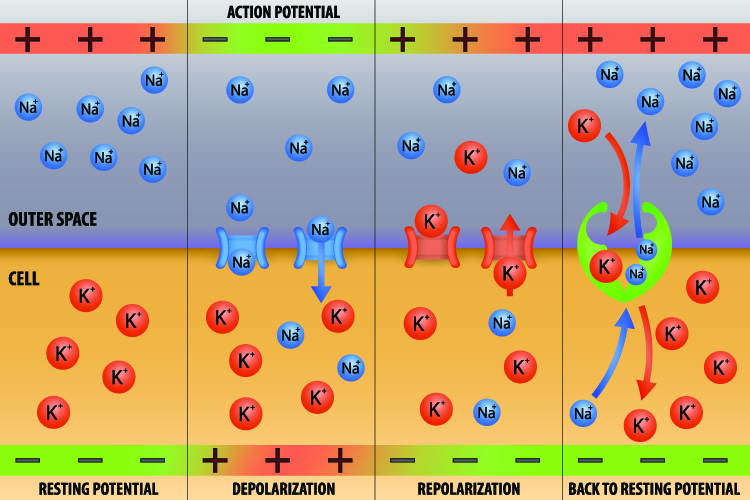
What is an Action Potential?
So, what is an action potential? Many definitions tend to be quite complicated, especially when you are learning about action potentials for the first time. To completely unravel the mystery, we should first look at the meaning of the term.
The word potential, in this case, does not mean the chance of achieving something, but refers to an electric potential. This is a continuous energy field more usually associated with the field of physics. In biology, potentials are found at the inner and outer edges of cell membranes. Potential energy is stored energy, that is why it is continuous. When a ball is still, it has potential energy. When a neuron is not firing, it has potential energy. Instead of saying a cell – or rather its membrane – has potential energy, we say is has a resting potential.
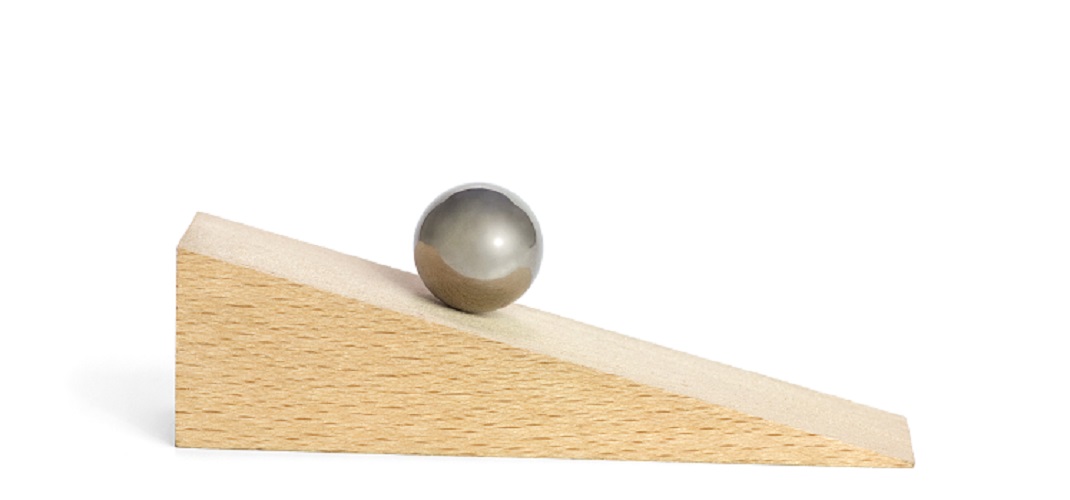
When force is applied to a ball, it will move. The potential energy changes into kinetic energy or the energy of motion. This force is not produced by the ball but comes from an external source; it replaces potential energy with kinetic energy. When this kinetic energy runs out (and no more external force is applied), the ball comes to a stop. It then possesses potential energy once more.
In the cell membrane, charged atoms called ions cause the equivalent of motion – they cause action. When a neuron is not firing and when a cell membrane is not allowing large amounts of certain products (we will talk about these later) to enter or leave the cell, that cell has resting potential. When electrical activity is stimulated, the potential stops resting because external forces create electrical movement – an action potential.
In order to fully understand this mechanism, we also need to know more about the electrical charge – the energy field – that is associated with a resting state and an action state. An electrical charge is the result of atoms. If you know about the form of an atom – a proton and neutron nucleus with a cloud of electrons circling this nucleus – you will probably know that protons are positively charged and electrons are negatively charged. This is just a fact; there is no need to understand why.
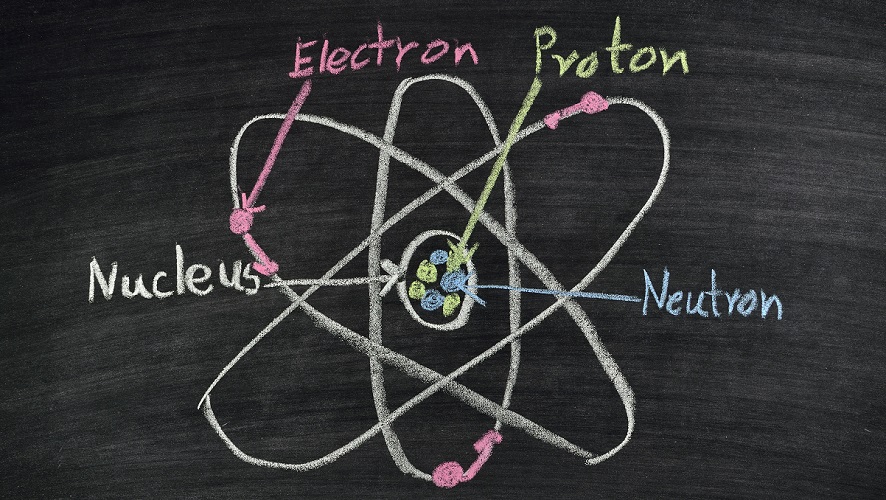
Usually an atom has the same number of positive protons as it does negative electrons. If it does not, it tries to join with other atoms so that these charges are similar and the atom can be stable. In a neutral state, an atom is just an atom. When it has too many electrons it will have a negative charge and is called a negatively-charged ion. If it has too few electrons (the number of protons stays the same), the positive charge of the proton is larger and the atom is called a positively-charged ion. These ions are extremely important when talking about electrical signaling in the form of action potentials. Cells that use action potentials are neurons and muscle cells.
Electrical Cell Membranes
Cell membranes are electrical. They use ions on either side of the cell – extracellular ions and intracellular ions – to create a charge that runs all the way along the cell membrane. When nothing much is happening the cell membrane is said to have a resting potential. Many products can enter the cell by diffusing through open pores or through the double phospholipid membrane. All cells have membranes with a resting potential. However, not all cells can produce an action potential.
Many particles need help to enter or leave a cell, including charged particles or ions. They require special channels that close and open. The different ways of entering and exiting a cell can be studied in the articles about passive transport and active transport; all you need to know in order to understand the action potential is that this is the swapping over from a resting state to an action state through changes in electrical charge caused by changes to the (resting) internal and external concentrations of ions.
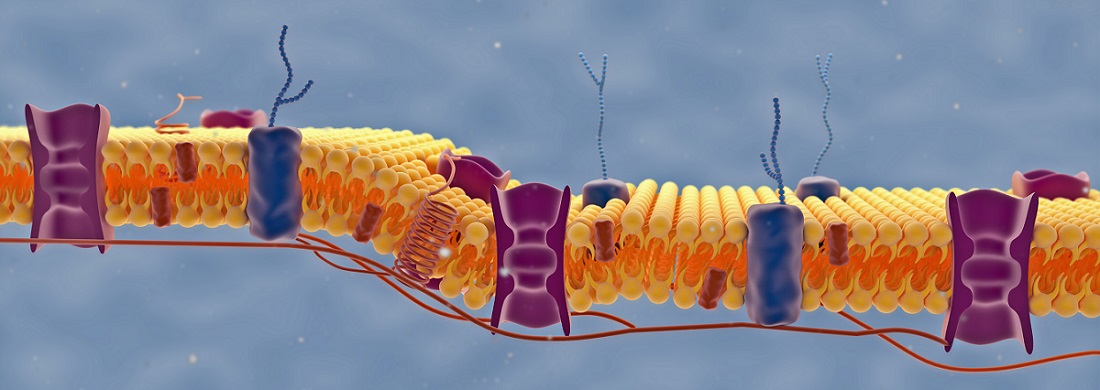
A membrane potential describes how an electrical charge is spread across the membrane. It is measured in millivolts (mV). This is most commonly measured by looking at the charge on the outside of the cell (the side where the extracellular fluid is) and comparing this with the charge on the inside of the cell (the cytosol or intracellular fluid). To keep calculations as simple as possible it is supposed that the outer side has zero mV.
Usually, the numbers of negative and positive ions inside the cell and in the surrounding fluid are similar and, therefore, neutral. However, in a region that lies extremely close to the membrane’s inner and outer surfaces a difference can be detected.
In a resting state – resting potential – the channels that allow charged particles to flow in and out of a cell are predominantly closed. There are very specific concentrations of ions close to the surfaces of the inner and outer membrane. You find more positive potassium ions (K+) inside the cell than outside; there are more positive sodium ions (N+) outside the cell than inside. Negative charges inside the cell are mainly composed of larger proteins called anions that cannot make their way through the ion channels in the membrane. So electrical signaling is the result of the movement of positive ions.
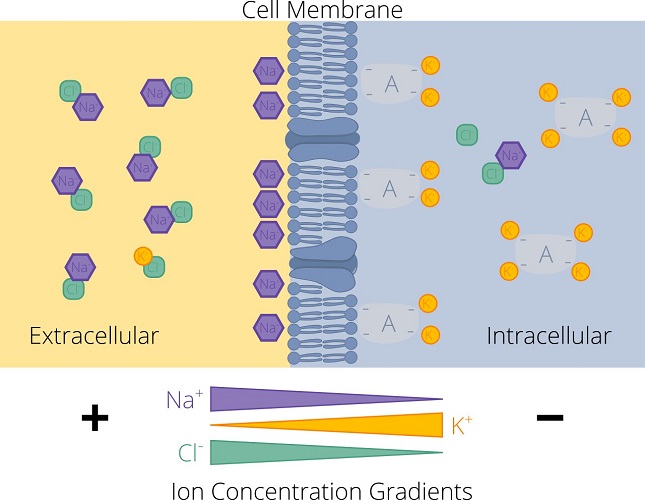
Ion Channels
Ion channels are necessary as we have already seen. This university site has published a song to help you remember a number different channel types. Ions are hydrophilic and cannot move through the lipids in a membrane. To move inside and outside the membrane they need specially-shaped proteins that create tunnels or channels. Channels that transport ions are called, not surprisingly, ion channels. If an ion attaches to an ion channel, the form of the protein channel changes shape and the attached ion can move through it. Alternatively, stretching of the cell membrane (stretch-gated channels) or differences in cell membrane voltage (voltage-gated channels) can stimulate these channels to open. If a protein is necessary to open the channel, this channel is ligand-gated. Each channel type has subtypes. This means a single neuron membrane may have around ten different types of ion channel.
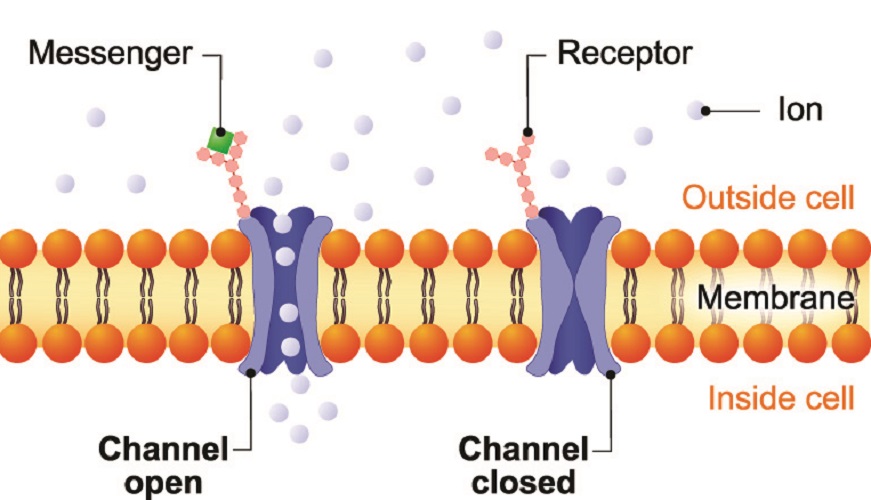
Up to one hundred million ions will pass through a single ion channel every second. This would usually requires a lot of energy, but the body saves this energy requirement by also letting the ions travel through a concentration gradient when at rest. If there are too many Na+ ions outside the cell membrane, they will travel to the inside of the cell via non-gated leak channels that provide a form of passive transport – passive transport does not require an energy source. The greater the difference in concentration on either side of the membrane, the quicker the flow of ions. As concentrations become closer to normal, the rate slows.
Another method is the sodium-potassium pump that works during both resting potential and action potential phases, shunting out three sodium ions and bringing in two potassium ions each time. This is an active transport mechanism that requires ATP.
But both sodium and potassium ions have positive charges, so how can this create electrical activity? The inside of the cell contains numerous anions – negative atoms and larger molecules (proteins) that usually stay where they are – the larger molecules are too big to get out. If you want to change the negative charge inside the cell membrane, you let positive ions from outside of the cell flow in. When you want to return to a negatively-charged resting potential, you let the positive ions flow out of the cell. The negative charges inside the cell stay put.
Negative and positive charges, as we know, can attract and repel. This is of importance in the action potential, as then we add the movement of this change in electrical charge to the mix. Then the attraction between negative and positive provides pulling power to allow voltage changes to travel in one direction – an impulse.
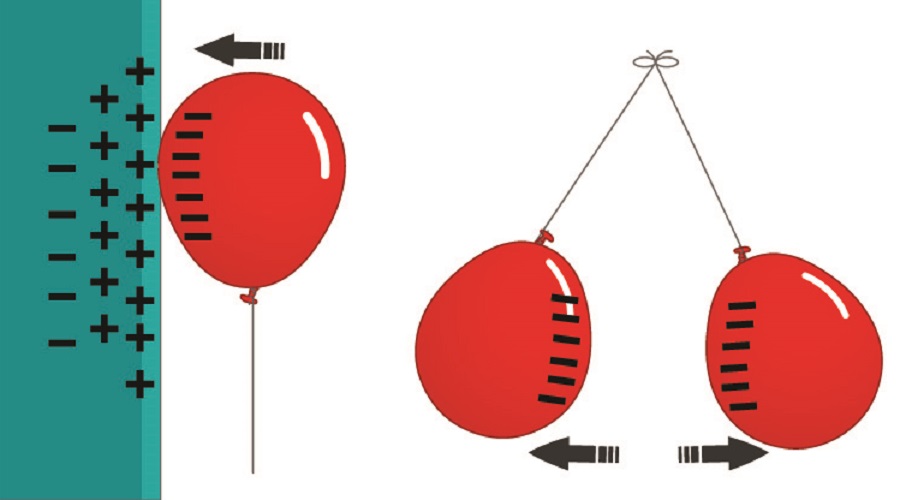
Action Potentials in Neurons
Actions potential examples in neurons include all types of sensory and motor messaging. Without action potentials we cannot function. The nervous system collects information from our senses, processes it, and sends further information that makes us respond. Every message travels by way of an action potential sent along the membrane of a neuron. The action potential is an electrical stimulus that might activate another cell to produce a hormone, or to release a neurotransmitter, or move a muscle. Action potentials are anatomical telephone wires and Ethernet cables from the time preceding wireless communications.
Neurons can be very long. The longest neuron axon in the human body runs from the bottom of the spine to the big toe. A blue whale has a dorsal root ganglion that runs from the spine to the brain and is longer than sixteen meters. The recurrent laryngeal nerve runs all the way along the neck of a giraffe – around two and a half meters. The change in membrane potential must somehow continue. This is done by changing from resting potential to action potential in stages, keeping the electrical message going until it reaches the end (synapse) of the nerve and pass on the message to the next cell.
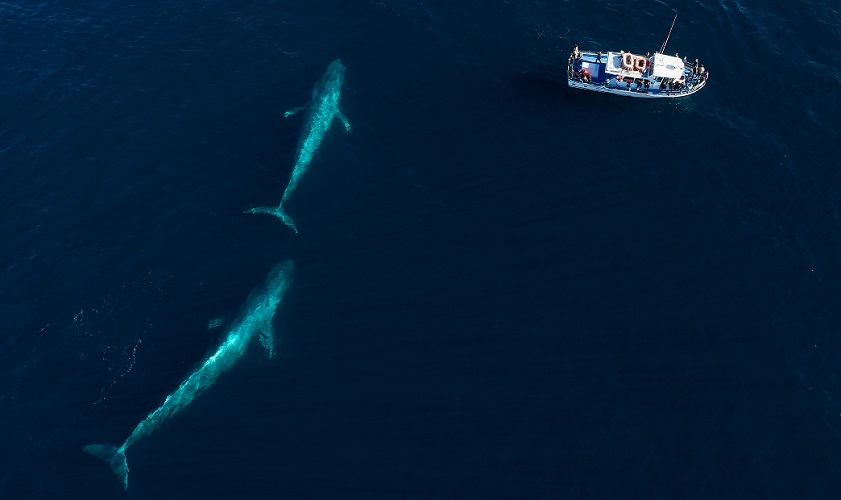
Neurons communicate electrochemically. They need chemicals (neurotransmitters) to initiate, speed up, slow down, and stop an action potential. They also need ions. All electricity generated in a living organism is electrochemical energy.
In the human body – and in the nervous systems of most mammals – the primary ions are sodium and potassium (both with a single positive charge), calcium with two positive charges (Ca2+), and chloride with one negative charge (Cl–). Chloride is an anion that is used to slow down action potential firing rates in some nerve pathways.
Resting Potential vs Action Potential
The difference between resting potential and action potential rests primarily on the difference in inner membrane voltage. The resting potential of a nerve cell is approximately -70 mV. Remember that the outside of the membrane is calculated as zero – so we can see that the anions outnumber the positively charged ions inside the cell. The membrane of a resting neuron or muscle cell is always negative. Additionally, there are more Na+ ions on the outside of the cell than inside, and more K+ ions on the inside of the cell than in the extracellular fluid when the neuron or muscle cell is in its resting state.
So what makes a resting potential become an action potential? A good action potential example is your sense of smell. Can you smell anything right now?
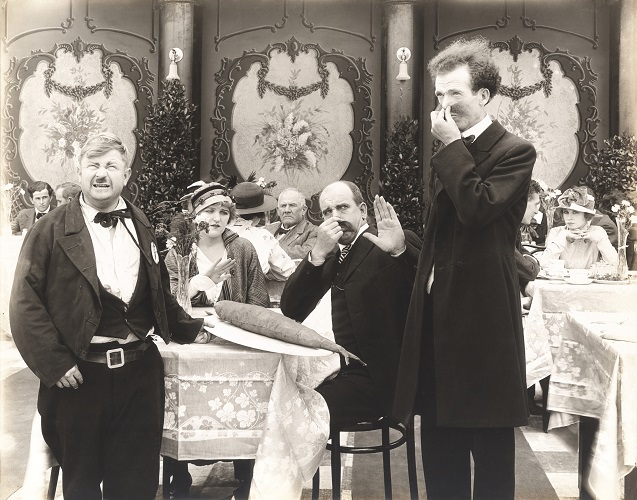
The chances are, you can’t. That does not mean your nose is not working, but you have probably been sitting in the same place while learning about action potentials. Perhaps, when you first sat at your desk or laptop, you noticed the smell of rain in the air through an open window or yesterday’s pizza. Your nose picked up odor particles via receptor cells and these receptor cells are the first step in the creation of an olfactory action potential. They either form an action potential themselves or release chemicals that cause a directly connected neuron to start the process. This process is called sensory transduction.
So what initiates an action potential? Imagine that, when you first stepped into your study area, the receptor proteins scattered throughout the surface of your olfactory cilia cells bonded to gaseous bubbles of old pepperoni pizza. These receptors activate and release a protein called G-protein. Via another chemical, G-protein increases the concentrations of another protein called cyclic adenosine monophosphate (cAMP). High concentrations of cyclic AMP tell the sodium ion channels of the cilia cell to open.
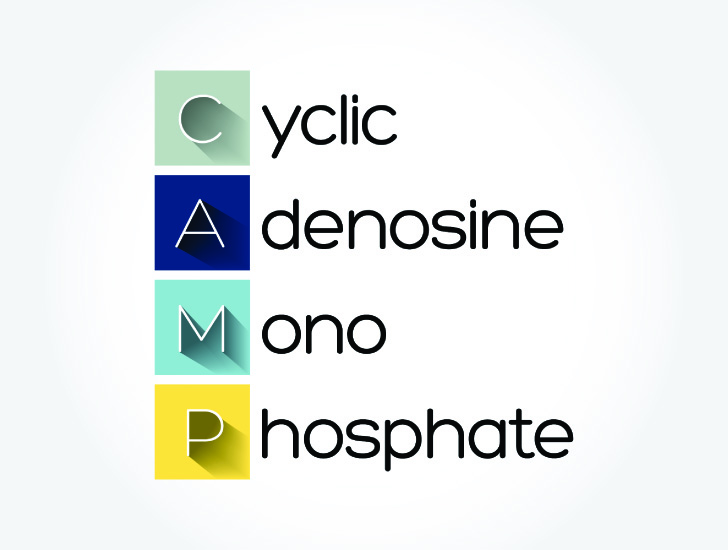
Positively-charged sodium ions rush into the cilia cell. The inside of the cell membrane is no longer -70 mV and climbs. The period in which the inside of the cell becomes more positively charged is called depolarization – an extremely important term you should be familiar with. Memorize depolarization as a change in electrical charge on the inside of the cell, very close to the membrane, and that this new charge becomes more positive than the ‘at rest’ charge.
As the charge passes a threshold of -55 mV, an action potential can initiate. If the charge only reaches -65 mV before the receptor cells stop responding, an action potential will not fire.
There have to be enough positively-charged ions inside the cell to cause an electrical effect – in biology terms the cytoplasm at the inner side of the cell membrane must reach a threshold of approximately -55 mV to -50 mV before an action potential can occur. The depolarization is now high enough to cause an effect.
Depolarization takes place from the moment the resting potential starts to climb (for simplicity’s sake we will say from -69 mV) and stops when the membrane potential reaches a peak of +30 to +40 mV. Unlike some sources might indicate, depolarization is not an action potential. It simply describes an increase in positive charge.
The threshold is reached, sodium ions continue to flow in, and depolarization continues as an action potential is fired. It is also described as an impulse, a spike, or a message. An action potential is always the same size. It is not more powerful or bigger when the inside of the cell membrane receives a larger influx of sodium ions. The message will not increase in importance if the threshold is exceeded. The size of an action potential is constant. Either the threshold is reached and an impulse initiates or it does not – the ‘all or none’ principle.
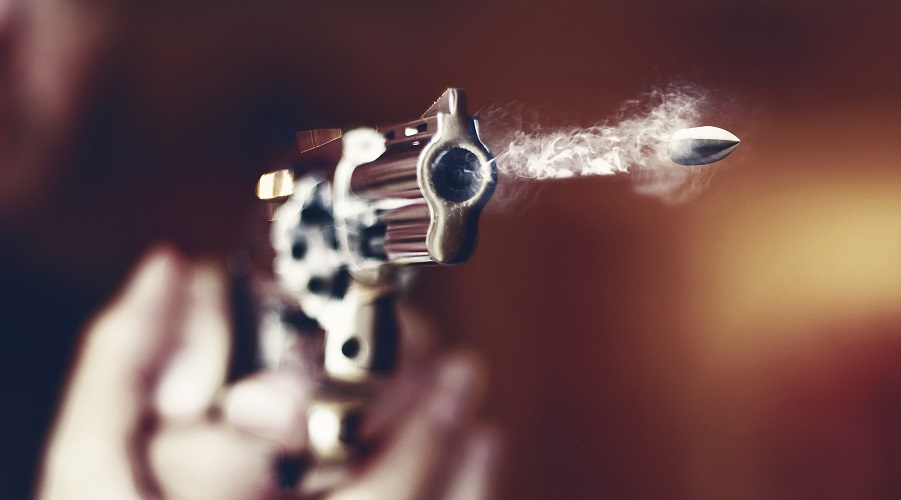
The only reason we tend to respond more to certain stimuli is due to the rate of firing, not the size of the action potential. In our example, when you first arrive in your study area you may notice the odor of stale pizza because the firing rate of the cilia action potentials was rapid. The detected smell was new. After a time, the cilia receptors experience temporary sensory fatigue. They stop detecting the old smell and start concentrating on new ones. If nothing is as strong as that old pepperoni pizza, you won’t be aware of any odor at all. The action potentials have not become smaller, they are either there or they are not. The cilia simply stopped responding to one odor and depolarization stops. If your nose detects the smell of burning, you will continue to notice the smell for a much longer period of time – a fire is dangerous and a pepperoni pizza is not.
Action Potential Propagation
Action potential propagation describes how an impulse moves along a cell membrane, most commonly the axon of a nerve cell. We already know that many neurons are incredibly long. In order to ensure an action potential continues without being lost or without the amount of depolarization being reduced to below threshold (some ions will continue to move out of the cell via leakage channels), the action potential needs to continue along the axon. To make this as efficient as possible in neurons that do not have an insulating myelin sheath, sections of the cell membrane depolarize at a time, pulling the action potential in one direction towards a target cell. This section-by-section movement is action potential propagation. First initiation, then propagation.
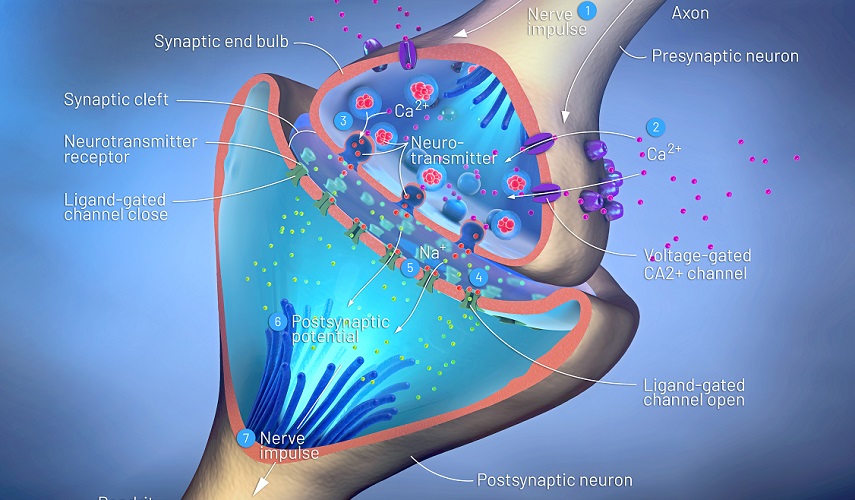
Think of a single ion channel inside a membrane that has received a message from cAMP to open and let sodium ions into the cell. If the nerve cell in question is located in the neck of the giraffe, how does it keep enough sodium flowing in and stay above the threshold levels as it moves along? While more than one ion channel will be affected by cAMP, how will the action potential move in one direction all the way to the nerve cell synapse? This is where negative and positive attraction comes in.
As sodium ions flow in, the area of the cell membrane close to the ion channel will start to become more positive. It takes time for atoms to diffuse completely into the cytoplasm and a nerve needs lightening speed. In the short space of time it has been given, the threshold charge only exists slightly to the left and right of the ion channel. Imagine a single plus under an ion channel surrounded by minus signs.
Due to positive and negative attraction, that plus is pulled in the direction of the next ion channel; it is a positive charge, so it slightly neutralizes the negative charge that attracts it. The region immediately under the first ion channel has now lost its positive charge by passing it along and becomes negative once more. Every time the positive charge arrives at the next point, the neighboring negative charge pulls it closer.
This change in voltage opens other sodium channels that do not rely on cAMP but on the change in charge – voltage-gated sodium channels. This way, any leakage of sodium ions is compensated for. An action potential is, therefore, like a string of light bulbs that are lit one at a time in a single direction, one after the other. The lighted bulb indicates the positive charge.
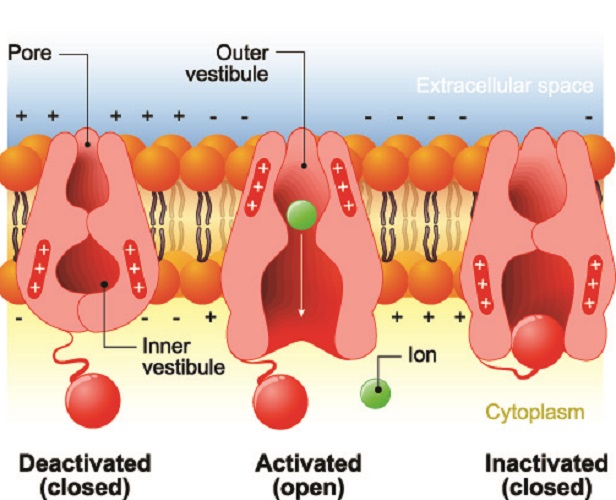
After depolarization comes repolarization. As we know that depolarization means an increasing (positive) difference in charge at the inside of the cell membrane, we can then surmise that repolarization is the opposite – the return of the inner membrane to its resting potential by returning to the original negative charge. If depolarization is an uphill line, the repolarization line points down. This can only happen with potassium ions. Voltage-dependent potassium ion channels open in the presence of a positive electrical charge inside the cell and help K+ ions to move out. At the same time, the sodium ion channels close. With all of these positive ions either leaving the cell or unable to enter, the resting potential is achieved.
In fact, the resting potential is completely passed by. At first, the electrical charge of the inner cell membrane becomes lower than the resting potential. When the charge is lower than -70 mV, we speak of hyperpolarization. This is because the sodium and potassium channels are unable to immediately close once -70 mV has been achieved.
In peripheral neurons, action potential propagation occurs at the nodes of Ranvier between individual Schwann cells. Schwann cells cover the axon of the neuron in myelin, a form of insulation that stops voltage-dependent sodium channels from letting sodium ions in. Even though this insulation layer also reduces leakage, the amount of depolarization would reduce as sodium ions diffused away from the immediate area. A nerve impulse would initiate but fade away.
In order to prevent this, unmyelinated nodes between the Schwann cells mean ion channels can let sodium ions in. In this case, each node of Ranvier is a light bulb with a positive charge, sending the message along an axon. The inner positive to negative pull also ensures the action potential continues to travel forward.
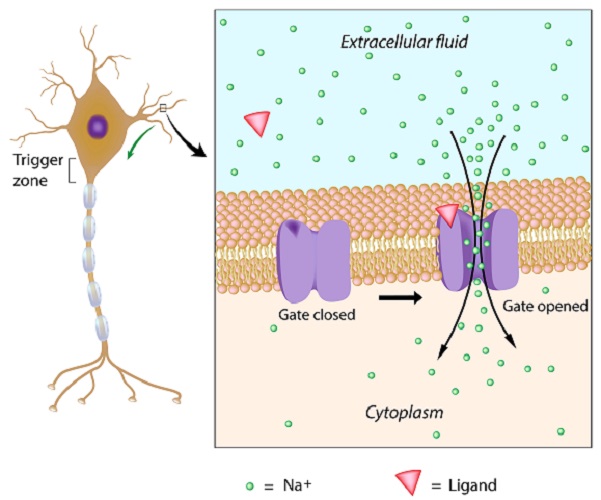
But how does an action potential travels in one direction? How is it that the pull of the next negative charge brings the action potential forward and not backward?
One-way action potentials are the result of the ion channel refractory period. Once an ion channel has opened, it cannot open again for a period of time – approximately one to two milliseconds. This is enough to ensure that the next voltage-dependent ion channel in the chain reacts to the change in charge and allows the next batch of Na+ ions to flow inside the cell, raising the charge at the inner membrane around that channel.
Skeletal Muscle Action Potentials
Action potentials cause contraction in muscle fibers. Each skeletal muscle fiber is a single cylindrical muscle cell and groups of hundreds or thousands of fibers (cells) form a muscle. You can see how a muscle is formed at the end of this section. Action potentials in skeletal muscle need more influx of sodium as the inner voltage of a muscle cell is around -90 mV.
In skeletal muscle, action potentials travel from the brain via a motor neuron. The neuron and muscle almost connect at the neuromuscular junction, with only a tiny gap (synaptic cleft) between them. When the action potential reaches the motor nerve ending it stimulates the release of acetylcholine, a neurotransmitter. Tiny molecules of this neurotransmitter cross the synaptic cleft and are detected by receptors on the muscle membrane.
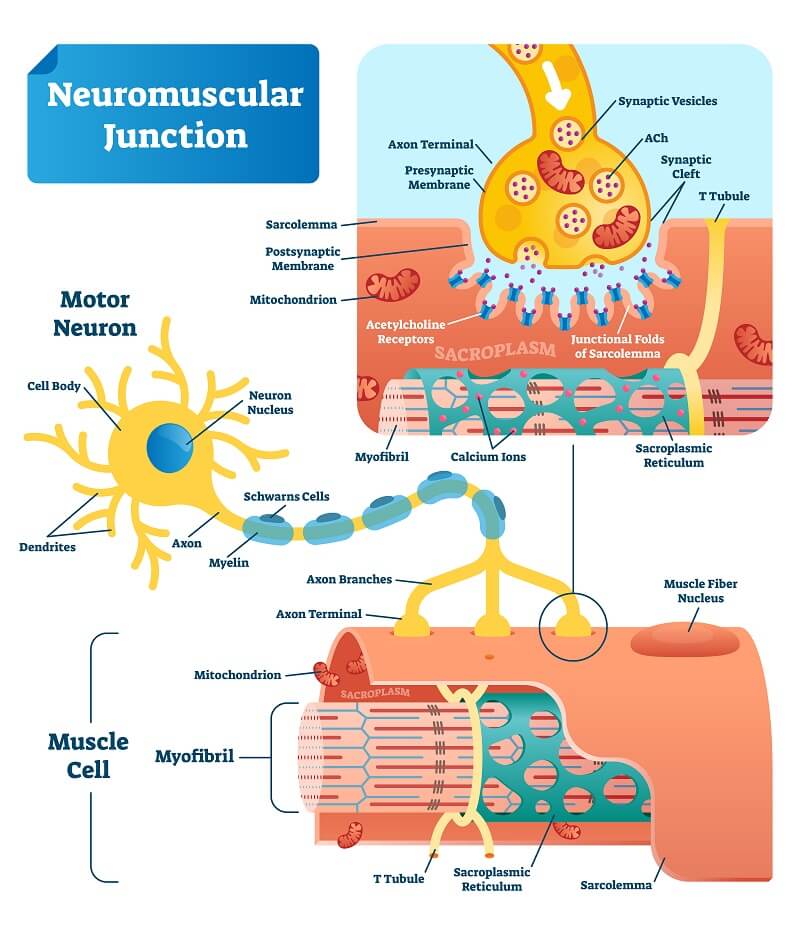
Muscle membrane ion channels open in reaction to the detection of acetylcholine by muscle receptors. Sodium ions flow into the cell; when the threshold is reached the muscle cell opens its calcium ion stores (Ca2+). It is calcium that enables muscle fibers to contract. When the action potential ends, the sodium ion channels close and the muscle cell can relax.
In skeletal muscle, action potentials do not affect one muscle cell. Instead, multiple muscle fibers or cells are connected to one motor neuron. When this single neuron sends action potentials to the muscle cells, they all react and contract. As a muscle contains many groups of muscle cells, each group wrapped in a membrane, more than one motor neuron is required necessary to cause it to contract. These motor neurons don’t fire at exactly the same time so that contraction remains constant – otherwise we would have very jerky movements as the muscles went back and forth in contract and relax mode.
Physiologists look at muscle movement by recording the electrical activity of action potentials on all muscle fibers at once. A compound action potential (CAP) is the result of multiple action potentials from multiple motor neurons on a single muscle or group of muscles and can be shown on a graph.
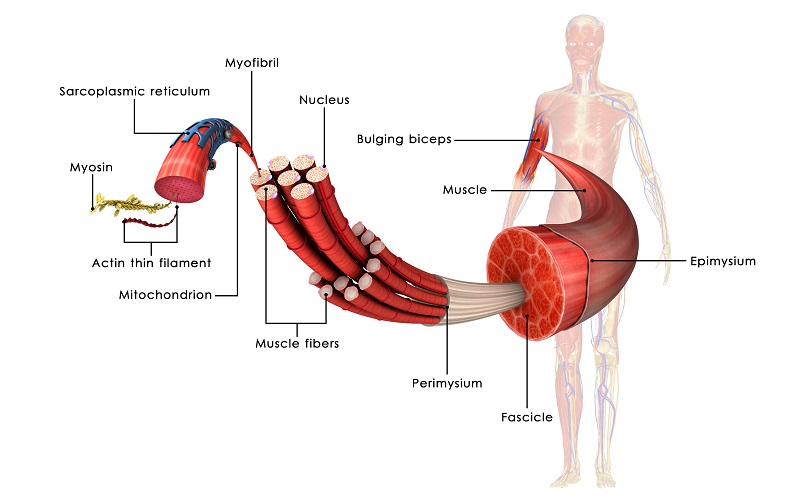
Cardiac Action Potentials
Cardiac action potentials involve heart cells. They are initiated in pacemaker cells that lie in areas on the inside of the heart muscle such as the sinoatrial or SA node. Action potential rates, dictated by our sympathetic and parasympathetic nervous systems, decide how quickly or slowly the heart beats. This is not a period of rest, as if the heart takes a break our body does not get enough oxygen.
It is at the cardiac action potential that many cardiovascular drugs have an effect. The image below shows the cardiac action potential graph (you will soon see that it differs from the neuron action potential graph), and also where different heart medications take effect. The cardiac action potential graph has four phases:
- Phase Four: diastole and pacemaker potential
- Phase Zero: depolarization (sodium and calcium ion influx)
- Phase One: slow repolarization – an extremely short phase of sodium ion gates closing and potassium gates opening
- Phase Two: slow repolarization – influx of calcium ions to aid with muscle contraction
- Phase Three: rapid repolarization
You can also note where these action potentials take place in relation to an electrocardiogram.
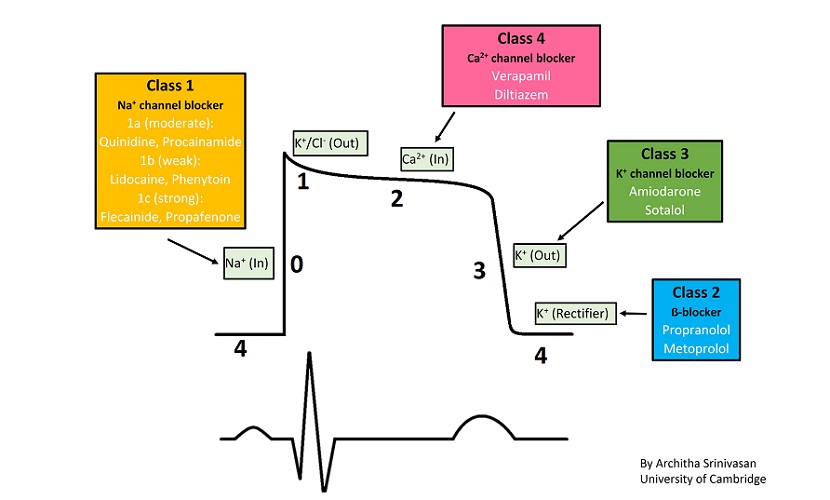
Pacemaker cells have an action potential phase called pacemaker potential that replaces the resting potential phase of nerve and skeletal muscle cells. This is because the heart is never at rest. As with the action potentials of skeletal muscles, more positive ion influx is required as the resting voltage at the inner cell membrane is -90 mV.
Action Potential Steps
Action potential steps can now be explained in short as the preceding information will have given you more insight into the meaning and mechanism of nerve impulses and cell membrane potentials.
The different action potential phases or steps are:
- Resting potential. Approximately -70 mV or -90 mV. All voltage-gated ion channels are closed. Leakage-gated ion channels and the sodium-potassium pump helps to keep the resting potential stable.
- A chemical stimulus, for example cAMP or a neurotransmitter, opens the sodium ion channels closest to this stimulus.
- Depolarization. The subsequent influx of positively-charged sodium ions into the cell causes a rapid positive change in voltage at the inner side of the cell membrane.
- Threshold potential. As the negative charge inside the cell membrane rises, it reaches a threshold voltage of approximately -55 mV. At threshold, voltage-gated ion channels are activated. Only if the inner voltage reaches this threshold can the next step take place.
- Action potential initiation. With the action potential threshold reached, the action potential initiates. If the threshold level is not attained, action potential initiation will fail.
- Action potential propagation
- In unmyelinated neurons. The action potential travels along the inner side of the membrane. Sodium ions continue to flood in via voltage-gated ion channels and the inside of each cell membrane section will reach approximately +40 mV.
- In peripheral myelinated neurons. Sodium ion channels are only able to contribute at the nodes of Ranvier as the myelin sheath stops any ion movement through the cell membrane.
- Repolarization. After the action potential has fired, the sodium ion channels close and the potassium ion channels that take this cation out of the cell open. The charge inside the cell becomes negative as these positive ions are sent away.
- Hyperpolarization. Ion channels do not immediately respond and positively-charged ions continue to exit the cell. The inner side of the cell membrane develops a higher negative charge than at the threshold potential value. The voltage will be between -71 mV and -75 mV during hyperpolarization (undershoot).
- Resting potential. During hyperpolarization, all voltage-gated channels close and sodium and potassium ions pass through the cell membrane via concentration gradients (leakage channels) and pumps to maintain the -70 mV inner voltage. We have returned to square one.
Action Potential Graph
An action potential graph should now make complete sense. These show the voltage on the inside of the cell membrane on the vertical axis (in millivolts) and the time in milliseconds on the horizontal axis.
In the graph below, you can see how the voltage changes during each action potential step. At –70 mV we know that the cell membrane is in a resting state – the flat line shows the resting potential. This voltage remains stable as potassium and sodium ions gently travel through the cell membrane on a concentration gradient.
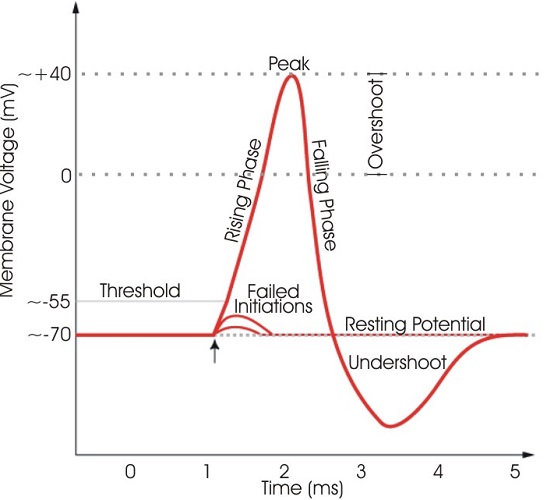
After stimulation causes sodium ion channels to open, the charge of the inside of the cell rapidly increases to a positive charge in the phase known as depolarization – the steep upward spike. Only after the charge has reached the threshold level of approximately -55 mV will an action potential follow.
Once the action potential initiates and propagates along the axon or cell membrane, negatively-charged potassium ions leave the cell and the sodium ion channels close. This is repolarization indicated by the steep downwards curve.
As the ion channels take a little time to respond, the inner side of the cell membrane becomes more negative than the resting value for a short time – hyperpolarization. As the ion channels respond, the voltage returns to -70 mV and the resting state returns.
Quiz