Transport Protein Definition
Transport proteins are proteins that transport substances across biological membranes. Transport proteins are found within the membrane itself, where they form a channel, or a carrying mechanism, to allow their substrate to pass from one side to the other.
The substances transported by these proteins can include ions such as sodium and potassium; sugars such as glucose; proteins and messenger molecules; and many more.
Transport proteins generally perform two types of transport: “facilitated diffusion,” where a transport protein simply creates an opening for a substance to diffuse down its concentration gradient; and “active transport,” where the cell expends energy in order to move a substance against its concentration gradient.
Function of Transport Protein
Life as we know it depends on the ability of cells to selectively move substances when they need to. Certain important molecules, such as DNA, must be kept inside the cell at all times; but other molecules such as ions, sugars, and proteins, may need to pass in and out in order for the cell to function properly.
Each transport protein is designed to transport a specific substance as needed. Some channel proteins, for example, open only when they receive the correct signal, allowing the substances they transport to flow on demand. Active transporters, likewise, can often be “turned on and off” by messenger molecules.
By moving substances across membranes, transport proteins make everything from nerve impulses to cellular metabolism possible.
Without transport proteins, for example, the sodium-potassium gradient that allows our nerves to fire would not exist.
Types of Transport Proteins
Channels/Pores
As suggested by their name, “channel” or “pore” proteins open holes in the membrane of a cell.
These proteins are characterized by being open to both the intracellular and extracellular space at the same time. By contrast, carrier proteins are only open to the inside or outside of a cell at any given time.
Channels or pores are typically designed so that only one specific substance can pass through.
For example, voltage-gated ion channels often use charged amino acids, spaced at precise distances, to attract their desired ion while repelling all others. The desired ion can then flow through the channel while other substances cannot.
Voltage-gated ion channels are good examples of transport proteins that act as needed. Often found in neurons, voltage-gated ion channels open in response to changes in a membrane’s electrochemical potential.
When closed, the voltage-gated channel does not allow ions to pass through the cell membrane. But when open, it allows huge quantities of ions to pass through very quickly, allowing the cell to change its membrane potential rapidly and fire a nerve impulse.
Carrier Proteins
Carrier proteins are transport proteins that are only open to one side of the membrane at once.
They are often designed this way because they transport substances against their concentration gradient. Being open to both sides of the membrane simultaneously might allow these substances to simply flow back along their concentration gradient, canceling out the carrier protein’s work.
To accomplish their work, carrier proteins typically use energy to change shape.
The sodium-potassium pump, for example, uses the energy of ATP to change its shape from being open to the intracellular solution, to being open to the extracellular solution. This allows it to collect ions inside the cell and release them outside of it, and then vice versa.
Other carrier proteins may use other energy sources, such as existing concentration gradients, to accomplish “secondary active transport.” This means that their transport is made possible through the cell expending energy, but the protein itself does not use ATP directly.
How is this possible? These carrier proteins often use the energy of one substance that “wants” to move down its concentration gradient in order to change its shape. The same shape change allows it to transport a substance that “doesn’t want” to move at the same time.
A good example is the sodium-glucose transport protein which uses the concentration gradient of sodium – originally created by the sodium-potassium pump – to move glucose against its concentration gradient.
We discuss the sodium-potassium pump and the sodium-glucose transport protein in detail below.
Examples of Transport Proteins
The Sodium-Potassium Pump
The most famous example of a primary active transport protein is the sodium-potassium pump. It is this pump that creates the ion gradient that allows neurons to fire.
The sodium-potassium pump begins with its sodium binding sites facing the inside of the cell. These sites attract sodium ions and hold onto them.
When each of its three sodium binding sites has bound a sodium ion, the protein then binds to a molecule of ATP, and splits it into ADP + a phosphate group. The protein uses the energy released in that process to change shape.
Now, the sodium binding sites are facing the extracellular solution. They release the three sodium ions outside of the cell, while the protein’s potassium-binding sites bind to two potassium ions.
When both potassium-binding sites are full, the protein reverts to its original shape. Now the potassium ions are released inside of the cell, and the empty sodium binding sites can bind more sodium ions.
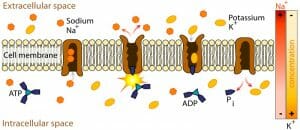
For each ATP this pump uses, it transports three positively charged ions outside of the cell, while transporting only two back into it. This creates an electrochemical gradient, with the inside of the cell being negatively charged relative to the outside solution. It also creates a strong concentration gradient, with much more potassium inside the cell and much more sodium outside of it.
When the time comes for a nerve cell to fire, the strong electrical and chemical gradients allow the cell to produce a huge, instant change by opening its voltage-gated ion channels.
Sodium-Glucose Transport Proteins
The sodium-glucose transport protein uses secondary active transport to move glucose into cells. They are active in intestinal cells and kidney cells, both of which need to move glucose into the body’s systems against its concentration gradient.
This operation requires energy, because the cells in question have a higher concentration of glucose than the extracellular fluid. Therefore, it would be impossible for glucose to diffuse into the cells on its own; energy must be applied.
In this case, the energy comes from the concentration gradient of sodium. Thanks to the action of the sodium-potassium pump, there is much more sodium outside of the cell than inside of it. There is a strong concentration gradient, then, favoring the movement of sodium into the cell.
This concentration gradient can be thought of as a type of “stored energy.” The sodium-potassium pump takes energy from ATP and turns it into this concentration gradient, which can then be used for other purposes, such as the sodium-glucose transport protein.
Gated Ion Channels in the Cochlea
Gated ion channels are passive transport proteins that open in response to specific stimuli. You may be familiar with voltage-gated ion channels, such as those that cause our neurons to fire in response to input from other neurons.
Less well-known are the gated ion channels of the cochlea – which are opened by mechanical pressure instead of voltage changes. These remarkable ion channels allow the nerves of our inner ear to fire in response to the vibrations of sound. This is how we hear.
In the cochlea, special cells called “hair cells” are responsible for our hearing. “Outer hair cells” sway in response to sound waves, amplifying their vibrations.
Inner hair cells, on the other hand, have a very special job. In response to these vibrations, they open ion channels in their cell membranes and release neurotransmitters – just like a neuron would.
Those neurotransmitters cause the firing of adjoining nerves. And that is how sound is converted into neural impulses!
Related Biology Terms
- Active Transport – Transport of substances across biological membranes, which requires the cell to expend energy.
- Gated Ion Channel – A protein that allows ions to pass through when open, which opens in response to a specific stimulus.
- Passive Transport – Transport of substances across biological membranes which happens naturally, without the requirement to expend energy.
Quiz
1. Why is it called “facilitated diffusion?”
A. Because the substance diffuses naturally down its concentration gradient, with no help from a transport protein.
B. Because the substance requires a transport protein to expend energy in order to facilitate its movement.
C. Because the substance diffuses naturally down its concentration gradient, but is helped by a protein that opens a channel or pore in the cell membrane through which it can pass.
D. Because the substance tries to diffuse, but is stopped by the cell membrane.
2. What is the difference between primary and secondary active transport?
A. Primary active transport uses carrier proteins, while secondary active transport uses channel proteins.
B. Primary active transport can only transport one substance at a time, while secondary active transport can transport two.
C. Primary active transport requires energy; secondary active transport does not.
D. Primary active transport proteins use ATP directly. Secondary active transport proteins use energy that is derived from other ATP-dependent processes.
3. Which of the following is NOT an example of active transport?
A. The sodium-potassium pump moves sodium and potassium both against their concentration gradient.
B. The ion channels of hair cells open in response to pressure, allowing ions to flow through.
C. The sodium-glucose transporter uses the concentration gradient of sodium to move glucose into the cell.
D. None of the above.
References
- Hall, John E.; Guyton, Arthur C. (2006). Textbook of medical physiology. St. Louis, Mo: Elsevier Saunders. ISBN 0-7216-0240-1
- Lever, J. E. (1992). Cell and Molecular Biology of Na /Glucose Symport. Membrane Transport in Biology, 56-72. doi:10.1007/978-3-642-76983-2_2