Definition
A neuron is a single nervous system cell that receives, processes, and transmits electrochemical messages from and to other cells. Neurons connect different areas of the central and peripheral nervous systems. Stimulated at one end by electrical or neurotransmitter activity, a change in membrane charge is initiated and sent as an electrical pulse (action potential) along the length of the nerve cell. This releases neurotransmitters at the end of the neuron that stimulate a response in the next cell. Neurons fall under various categories; however, all types of neurons function as electrochemical messenger cells.
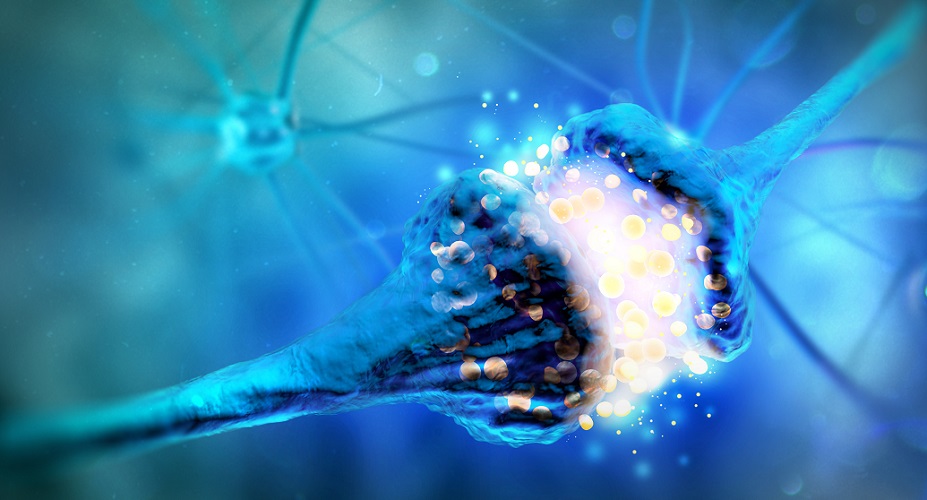
Parts of a Neuron
The parts of any neuron are the same, although how long these parts are or how many individual components they have differs according to neuron type. We can safely say that all neuron structure consists of a cell body, axon, dendrites, axon hillock, and terminal.
Neuron Soma
The main body of a neuron – the soma – contains intracellular structures called organelles that include the nucleus, Nissi granules (protein synthesis units composed of rough endoplasmic reticulum and ribosomes), mitochondria, lysosomes (digestive enzyme capsules), endosomes (vesicles that bring products into the cell), peroxisomes (vacuoles containing hydrogen peroxide reducing enzymes), separate smooth and rough endoplasmic reticulum around the nucleus, and multiple Golgi apparatus. Like most cells, these organelles sit within the cytoplasm which is surrounded by a cell membrane.
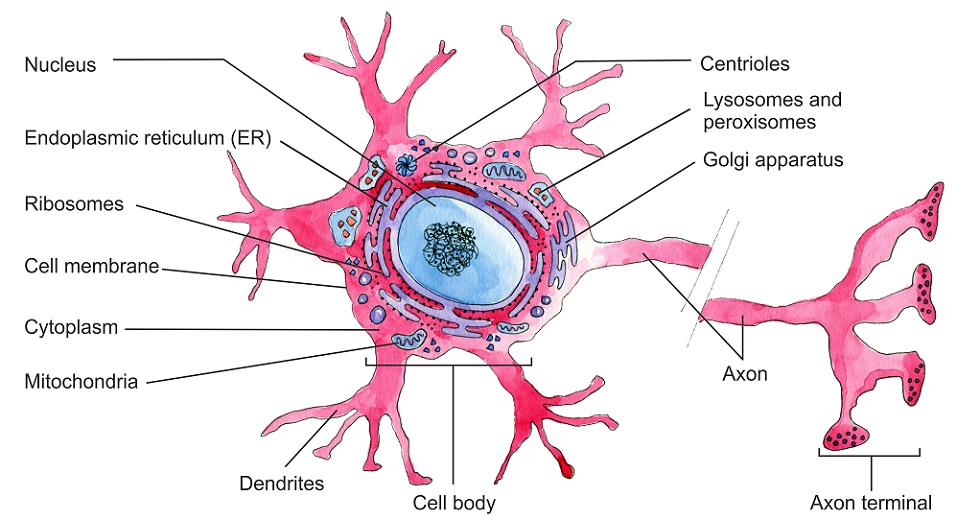
The neuron soma is where the dendrites (next section) attach, although signals are not transmitted here. The role of the soma is to provide energy for the cell to keep it alive and make repairs. Nerve cells are notorious for slow healing – this is because the soma must produce reparative proteins and then send them through often extremely long axons and dendrites.
Neuron Dendrites
Neuron dendrites begin at the soma and extend outwards, almost like the branches of a tree. Dendrites are the signal-accepting components of a nerve cell, receiving signals and passing them down towards the axon hillock (next section). Every branched end contains a synapse that connects with a preceding neuron or sensory receptors located in structures such as the retina of the eye, the lining of the gut, and the mucus membranes of the nose.
Some neurons have a single dendrite, others have many. Dendrites can be short or long. If a neuron only has one dendrite, it will synapse at a single point. Multiple dendrites mean that direct connections can be made with up to a thousand other neurons.
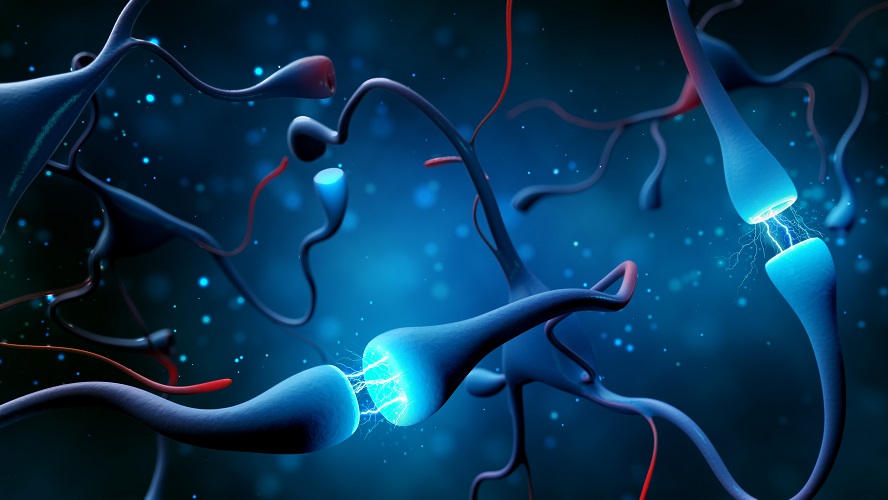
Dendrites contain well-ordered microtubules that transport substances to other parts of the cell. The further away a part of the nerve cell is from the soma, the more microtubules it contains. For example, neurotransmitters produced in the soma can travel up the microtubules to the axon ends where they are stored and used for synaptic transmission. Dendrites also contain rough endoplasmic reticulum and ribosomes. Many ligand-gated ion channels are found in the dendrite membrane. It is through charged ions that signals are carried; another molecule is required to open these channels to the influx and outflow of ions into the dendrite. This requirement for another molecule to open an ion channel is a characteristic of ligand-gated transporter proteins.
Axon Hillock
The axon hillock can be found at the point where the soma meets the axon; the neuron axon is described in the next section. Axon hillocks are extremely important as they initiate and regulate action potentials. Neuron action potentials – described in detail in another article and in less detail further on – are electrical impulses that travel along a nerve and allow signals to be passed on to target cell(s). Without action potentials, signals cannot be transmitted through a neuron and there will be no stimulus to produce an effect, such as muscle contraction or remembering that the shape and texture of the object you just saw is a dog. When the axon hillock receives a strong enough electrical stimulus from the dendrites it will fire an action potential that is carried along the axon.
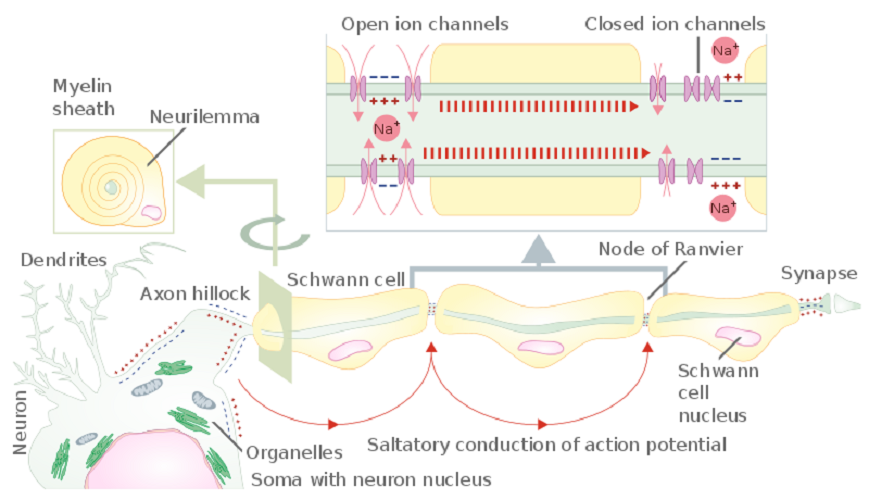
Our nerve cells are continuously in action. Some nerve cells are inhibitory, some excitatory. Some are modulators. Only when one type produces many more signals than the other will the axon hillock react. If the total number of excitatory signals reaches a certain threshold above the number of inhibitory signals, an excitatory action potential is triggered. Naturally, where more inhibitory signals are produced and these reach a certain threshold above that of the excitatory neurons, an inhibitory action potential will be initiated. In this way, gut peristalsis can increase or decrease, our breathing rate can change, and we can become tense or relax.
Neuron Axon
Neuron axons are the long, singular channels along which action potentials travel to reach the nerve cell end. The thicker the axon, the more rapidly the signal is sent. Some axons are covered with a white, fatty substance called myelin. White matter in the brain is white due to large quantities of myelinated axons. Myelin acts as an insulating coating just like the electrical wires of your phone charger are covered with a coating of rubber or plastic. The fatty layer stops electrical energy in the form of positively-charged ions (sodium, potassium, and calcium) from leaking out of the axon. Some sensory neurons are unmyelinated.
Many autoimmune diseases affect the quality of the myelin sheath. In multiple sclerosis, weak muscles and chronic pain are common. The spinal nerves that transmit information are unable to produce consistent action potentials as the damaged myelin negatively affects electrical activity.
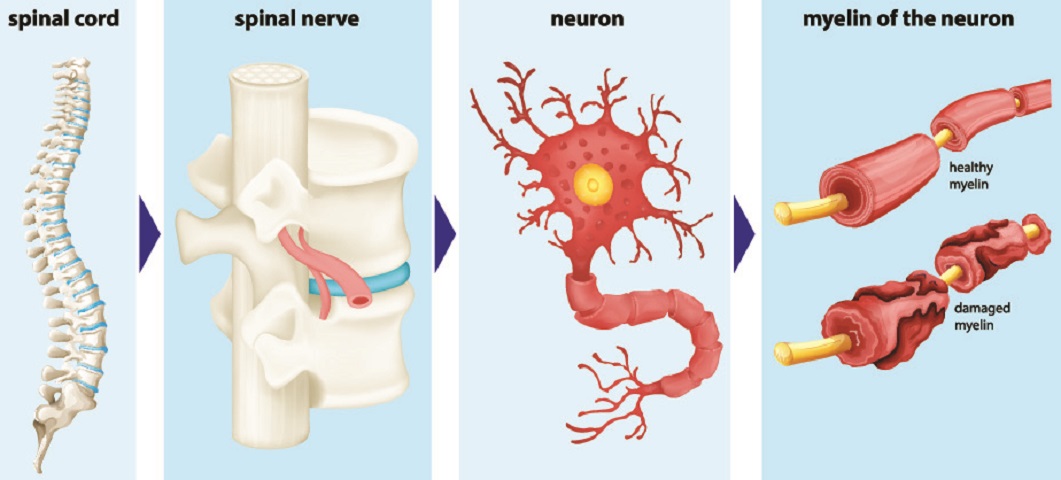
There are very few organelles in the neuron axon – mainly mitochondria to provide energy for opening active transport ion channels that are responsible for transmitting the neuron action potential. Axons can be very short – one-tenth of a millimeter – or extremely long. The longest axon in the human body is found within the sciatic nerve, running from the base of the spine to the foot.
Axons contain large numbers of voltage-gated ion channels that allow charged ions to flow in and out of the axon to keep the electric impulse moving along its length. Unlike ligand-gated ion channels that require other molecules to open them, voltage-gated ion channels open in response to changes in charge. As an action potential initiates at the axon hillock, the dendrites do not have voltage-gated channels.
Because myelin prevents anything from moving out of or entering the axon, these sheaths run in a bead-like pattern. Where each ‘bead’ meets the next is a small gap called the node of Ranvier. Here, the lack of myelin means that ions can keep the action potential strong enough to travel to the end of the nerve. You can clearly see the gap of the node of Ranvier between the dark-stained myelin sheath in the photograph below.
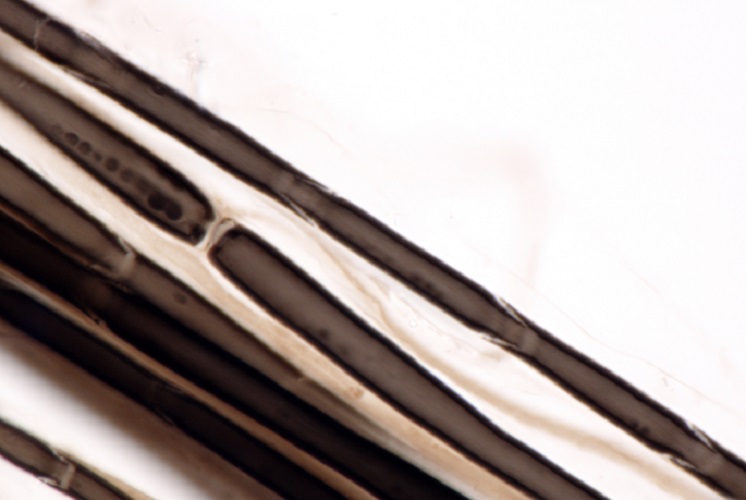
Neuron axons are always singular, but a single axon can split (bifurcate) to create two branches.
Neuron Terminals
Neuron terminals or nerve endings branch out from the end of the axon to form the neuron synapse – the meeting of the neuron with the next cell’s membrane. This part of the neuron cell is the presynaptic complement; the postsynaptic complement is found in the dendrite (where it receives information via neurotransmitters or electrical charge from the preceding cell).
At the end of the neuron terminals are boutons (terminal buttons). The terminal buttons of excitatory nerves are usually C-shaped and fit closely to the rounded dendrite of the next nerve – rather like a ball-and-socket joint. Inhibitory neuron terminal buttons tend to be flat and sit flush to the membrane of the next cell.
Neuron terminals have large numbers of mitochondria as they need to continuously release stored vesicles of neurotransmitters. These vesicles open at the terminal membrane, allowing released neurotransmitters to travel through the tiny gap (synaptic cleft) between the terminal and the receiving end of the next neuron cell. This is how an action potential creates a response in the postsynaptic cell. Terminal boutons or buttons also reabsorb any remaining neurotransmitters left in the synaptic cleft after a message has been sent on.
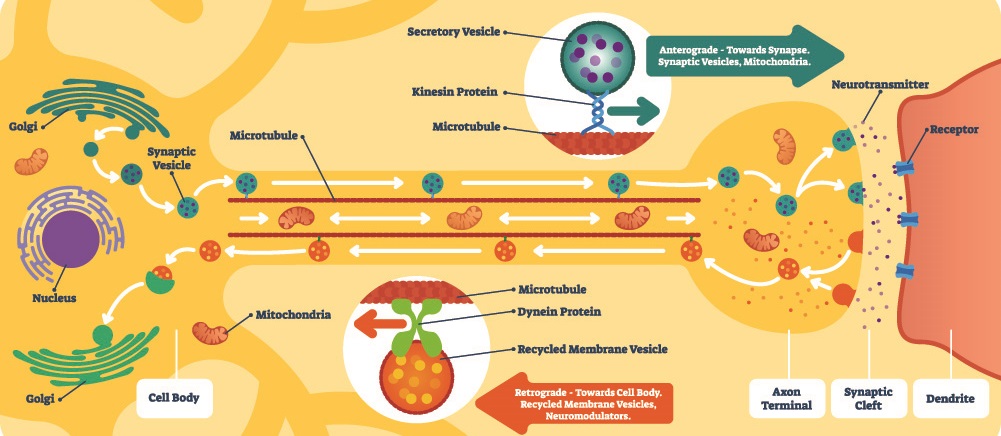
Neuron synapses can be chemical or electrical, although the majority are chemical. Chemical synapses are the result of neurotransmitter release and always travel in one direction. Electrical synapses are only possible when two cells are connected by channel proteins. The synapse can travel in both directions, although this is rare. A very limited number of neurons can communicate by way of an electrical field, without charged ions traveling through synaptic channel proteins.
An electrical synapse is much quicker than a chemical synapse. It does not depend on the availability of neurotransmitters and does not need to wait for neurotransmitter vesicles to open, or for the neurotransmitters to travel through the synaptic cleft, or for the next cell to absorb and respond to these neurotransmitters. The neurons of the hypothalamus use electrical synapses to secrete hormones. Some neurons in the hippocampus communicate via electrical fields.
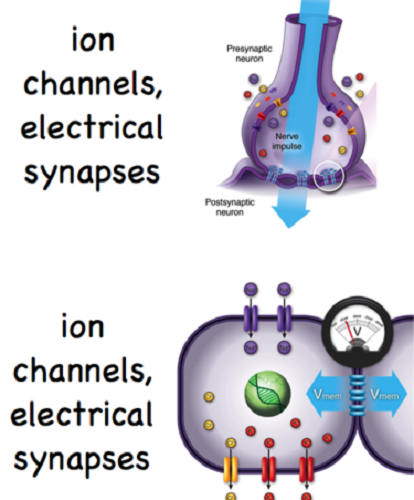
Neuron Categories
Neuron categories or neuron types can be split into five groups: unipolar, pseudo-unipolar, bipolar, multipolar, and anaxonic. If you are asked the question, “What is a neuron?” you are usually expected to describe the generic function – a cell of the central or peripheral nervous system that brings information from one part of the body by way of electrical impulses and chemical synapses. Perhaps you can mention the parts of a neuron and what these do. Listing the various neuron categories will probably earn you extra points.
When concentrating on human histology, we can immediately discard unipolar neurons as these are only found in invertebrates.
Pseudo-Unipolar Neurons
Pseudo-unipolar neurons are purely sensory neurons. As we have already seen, axons are always singular but they can split – bifurcate. This is obvious in pseudo-unipolar neurons as it looks like there are two axons to the left and right of the soma. This is one axon; one ‘branch’ of the axon connects to peripheral tissue and the other to the spinal cord.
Many textbooks say that pseudo-unipolar neurons have no dendrites – this is incorrect. The below image is a typical representation of the pseudo-unipolar neuron, but is confusing. The sensory receptor – the dendrite receptor – looks exactly the same as the terminal end which is not labeled.
The majority of examination questions will give an indication of the dendrite and terminal ends. If not, the dendrite end is usually closer to the soma, is not covered by myelin if the nerve cell is myelinated, and there may be some indication of Nissl bodies – granules of rough endoplasmic reticulum and ribosomes – that are found in the soma and dendrites but not at the axon hillock, axon, or terminals.
As the cell body of a pseudo-unipolar neuron always sits within a spinal ganglion (dorsal root ganglion), the receiving end (dendrite) is in contact with a cranial nerve or spinal nerve and the terminal connects to organs or tissues.
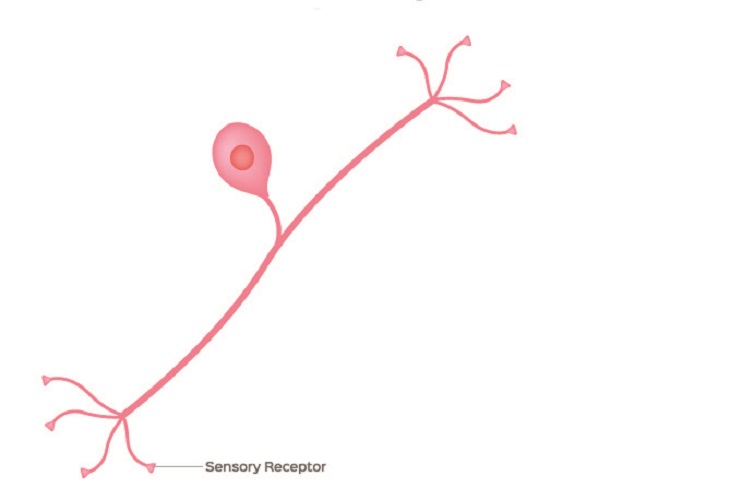
The term ganglia refers to groups of cell bodies. Ganglia are sensory and autonomic. Examples of human ganglia are the dorsal root ganglion, the paired ganglia of each cranial nerve (vagus nerve ganglia, trigeminal ganglia, and so on), sympathetic chain ganglia (of the sympathetic nervous system), and the autonomic terminal ganglia that regulate the functions of various organs. Where a ganglion is very close to the wall of the organ it innervates it is referred to as a plexus; however, a plexus is not characterized as a group of soma but as a nerve network.
Bipolar Neurons
Bipolar neurons are uncommon and limited to three regions – the retina of the eye (vision), the vestibulocochlear cranial nerve (hearing and balance), and the olfactory epithelium (sense of smell). These regions control the ‘special’ senses.
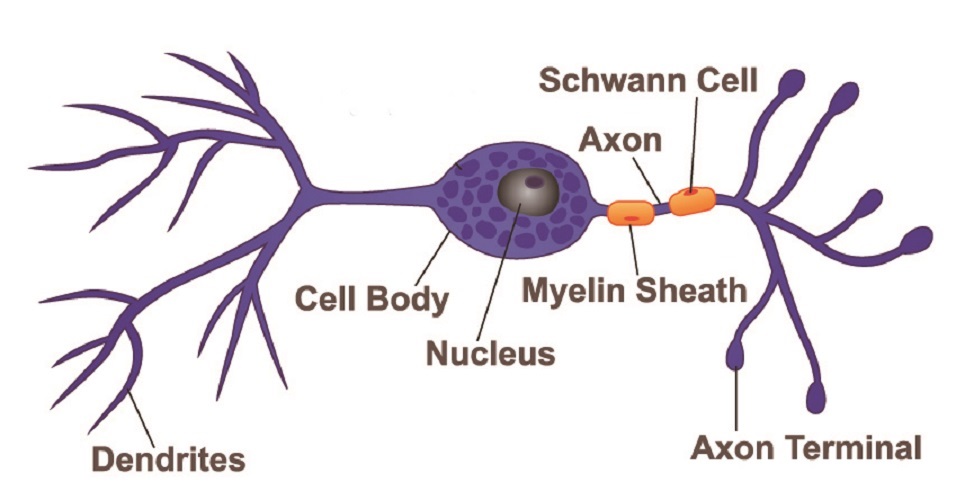
A bipolar neuron has the same structures as all neurons but is arranged differently. The central cell body makes a border between the dendrite end and the axon and terminal end. Dendrites are multiple and sometimes it is difficult to know which end is which in a simplified bipolar neuron diagram. Usually, the rounded shape of the terminal buttons and perhaps the presence of myelin will help you to figure out which end is which.
Multipolar Neurons
Most of our nerve cells are multipolar neurons. These cells have at least three protuberances coming out of the soma – one of these is the axon hillock and the rest are dendrites. Most of the neurons of the central nervous system are multipolar, such as motor neurons and interneurons. These categories are functional and are discussed in the neuron function section.
Unlike other nerve types, the soma also receives synaptic input, acting in the same way as a dendrite. The many dendrites mean that these neurons can pass on signals from multiple presynaptic cells.
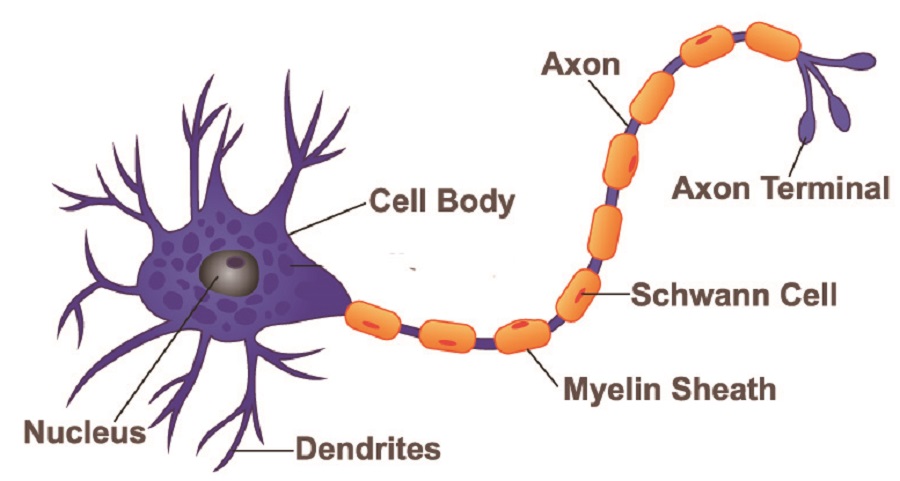
Anaxonic Neurons
The rare anaxonic neuron has no axon, either because the axon and dendrite function is indistinguishable (undifferentiated anaxonic), or because there is no axon as in the case of anaxonic periglomerular cells in the olfactory bulb and a handful of interneurons.
Granule cells in the central nervous system do not have an axon; however, they still produce localized action potentials. Because there is no axon, action potentials do not need to travel far and they are less likely to weaken. It is understood that the multiple dendrites of anaxonic neurons have receptor, conducting, and effector regions. Of all of the different types of neurons, we know the least about anaxonic neuron function. They seem to play more of a modulating role, just like interneurons.

Neuron Function
Neuron function is simple and very easy to learn. Neurons provide a means of communication between cells. Each nerve cell has three primary functions – to receive signals, to decide whether these signals should be forwarded, and to communicate approved signals to the next cell. Neuron parts have specific functions as discussed under the preceding heading.
Neurons are the electrical cables of the body. Think of the dendrites as the pins that enter a wall socket, the soma as the fused plug, the cable as the axon, and where the cable attaches to a device is the terminal.
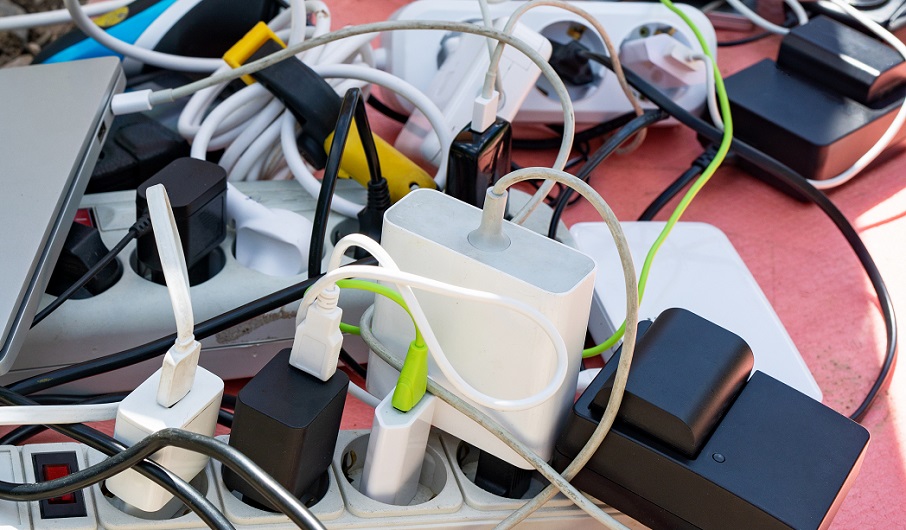
Nerve cell location does not affect neuron function – the functions always remains the same: reception, regulation, and transmission of electrochemical and electrical messages. However, neurons are grouped in various ways that can often make things confusing. We will have a look at the most common categories in the next paragraphs.
The primary neuron classification method distinguishes between CNS (central nervous system) neurons and PNS (peripheral nervous system) neurons. This category simply describes the location – either within the brain and spinal cord, or outside of the brain and spinal cord. Neuron function remains, as always, the same.
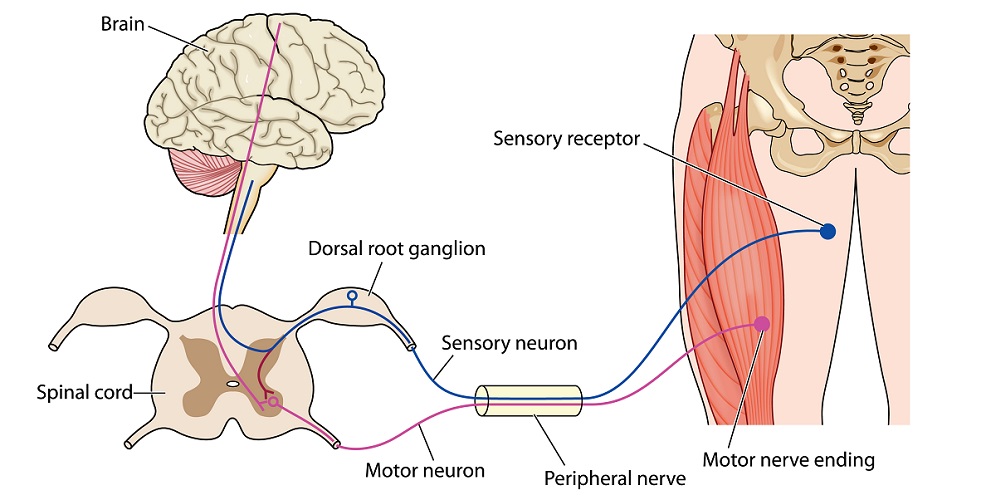
Another method of categorization is according to the type of information that is communicated – this method groups neurons into motor, (interneuron), and sensory functions.
CNS and PNS Neurons
In terms of CNS and PNS, the difference is in the location. The central nervous system is composed of the brain and spinal cord. Everything outside of these two structures is part of the peripheral nervous system.
Neurons in the CNS enable information to be analyzed and organized. Neurons in the temporal lobe will process different types of information compared with the neurons of the parietal lobes; they enable different lobes to communicate with each other, for example.
The PNS gathers sensory information and sends it to the CNS via sensory neurons (afferent neurons) and accepts and follows motor instructions from the CNS via motor neurons (efferent neurons).
For example, you trip up on an uneven stretch of sidewalk for the second time in the same week and graze your knee. Sensory nerve endings within the grazed skin tell the CNS that this area has been damaged. The CNS sends this sensory information to various regions of the brain that regulate how much pain you feel, increase blood flow to the area to bring in more infection-fighting white blood cells, tell the body to produce more blood clotting factors, remind you that you have already tripped over this section of sidewalk and should be more careful, and make you instinctively tense up and grab the damaged knee by forwarding instructions to your hands, arms, shoulders, and back via motor neurons.
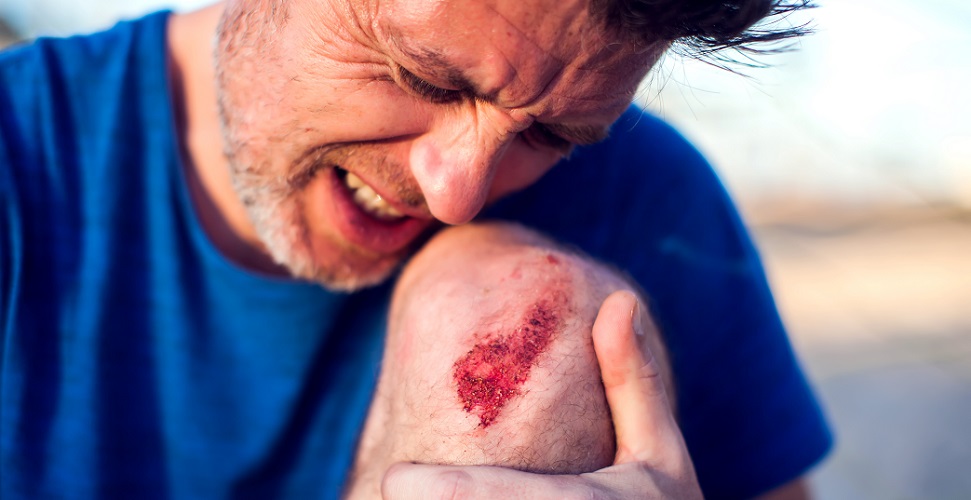
Sensory, Motor, and Interneurons
Neurons are also categorized according to the target tissues they communicate with. Sensory neurons are primarily pseudo-unipolar and found in both peripheral and central nervous tissue. They pick up information according to the five senses – smell, taste, hearing, sight, and touch, as well as data that helps us to know how our body is positioned and balanced (proprioception).
Spinal motor neurons are also part of the central nervous system (think of the knee-jerk reflex or how the muscles of your intestines work harder when you are resting). Upper motor neurons travel between the brain and spinal cord, and lower motor neurons between the spinal cord and muscle. A lower motor neuron always ends at a muscle fiber and is predominantly multipolar.
Interneurons or relay neurons connect a sensory nerve cell to a motor nerve cell as shown in the neuron diagram below. There can be more than one interneuron in a circuit.
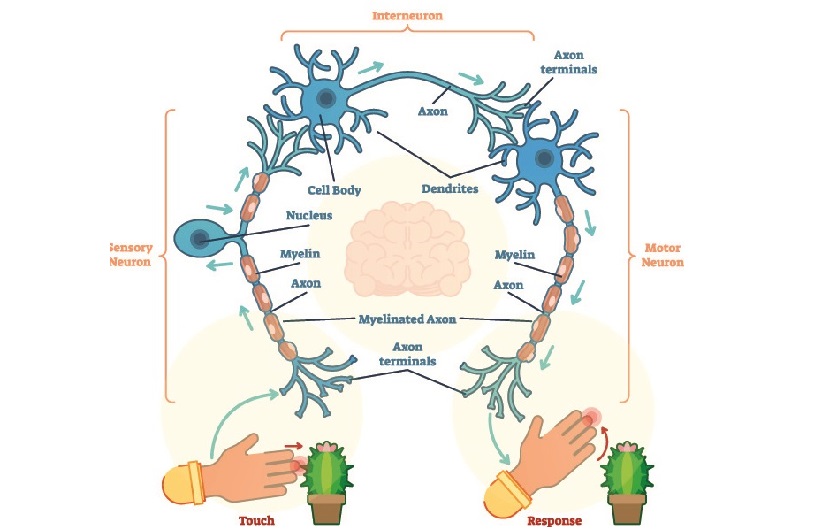
Interneurons are found in the spinal cord where they play a significant role in reflex arcs. In the cortex of the brain, interneurons seem to connect multiple regions to provide a complete set of patterns or behaviors – it has been noted that many psychiatric disorders show abnormal interneuron function. Current research has only managed to scrape the surface of the different cortical interneurons and we still have much to learn.
In the cerebellum, interneurons such as granule cells and unipolar brush cells (UBSs) regulate other functional neurons such as Purkinje cells that inhibit the firing rate of other neurons.
Autonomic Neurons
Autonomic neurons – naturally, part of the autonomic nervous system – react to sympathetic (fight/flight) and parasympathetic (rest/digest) stimuli that are not under our control. It is possible to stimulate one or the other system, however, by creating the right environment. For example, deep breathing during examination stress can counteract the body’s natural fight/flight reaction of a rapid heart rate, sweating, and muscle tension by stimulating the vagus nerve. The vagus nerve slows the heart rate and has a vast parasympathetic effect all over the body.
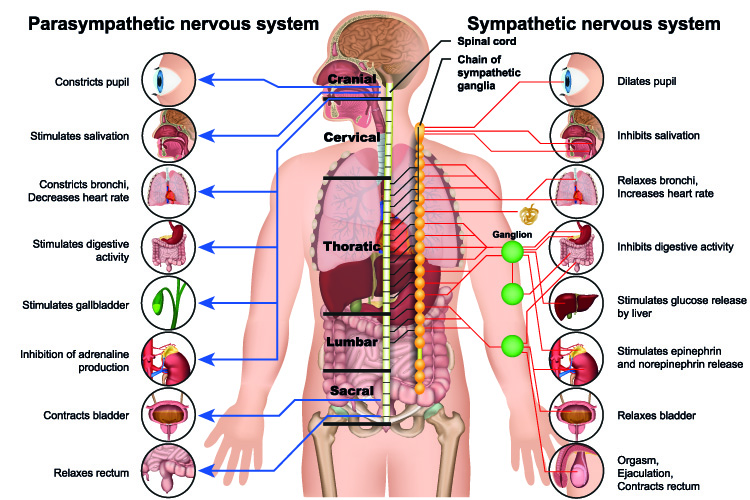
Specific to the autonomic nervous system, sympathetic and parasympathetic preganglionic neurons connect the central nervous system to autonomic ganglia. That means each impulse must cross a ganglion. Postganglionic neurons of the autonomic nervous system (when you see the word ganglion, you know that the autonomic or involuntary nervous system is involved) connect the ganglion to the target (effector) organ or cell.
This image shows the voluntary or somatic nervous system that controls skeletal muscle movement and the autonomic fight/flight and rest/digest divisions. You can clearly see that only the autonomic networks travel via ganglions.
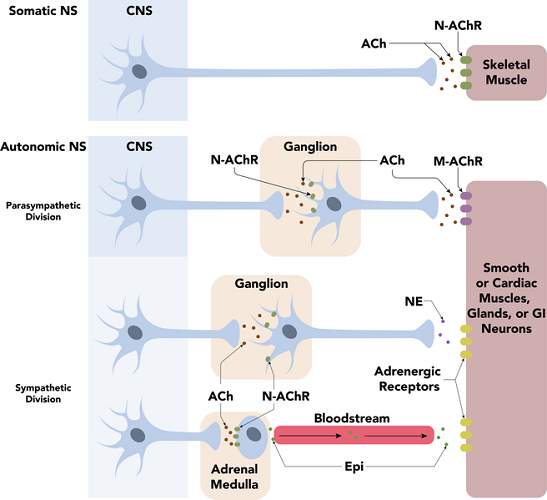
Neuron Potentials
Neuron potentials or action potentials will not be looked at in great detail here. It is important to know that action potentials practically always travel in one direction (some localized electrical action potentials can travel in both directions but this is rare) from dendrite or axon hillock to neuron terminal buttons.
Dendrites can be seen as the antennae – they pick up chemical (neurotransmitter) or electrical messages from preceding (presynaptic) cells. It does not matter if the neuron is a motor neuron, a sensory neuron, an interneuron, part of the CNS or the PNS, autonomic, or part of a voluntary neuron network – all behave in the same way.
Dendrites let charged ions flow into the cell membrane when helper molecules (ligands) open special ion channel gates (ligand-gated channels). They bring a very small electrical charge towards the soma.
At the axon hillock, the strength and frequency of the charge is measured. If strong enough – at threshold – an action potential is initiated. This is a change in the charge of a small section of the cell membrane that, thanks to the inflow of positively-charged sodium ions along the axon, moves in the direction of the neuron terminal. The axon is the cable whose sole function is to transmit the signal to the end of the line. It can be insulated (myelinated) or non-insulated (non-myelinated).
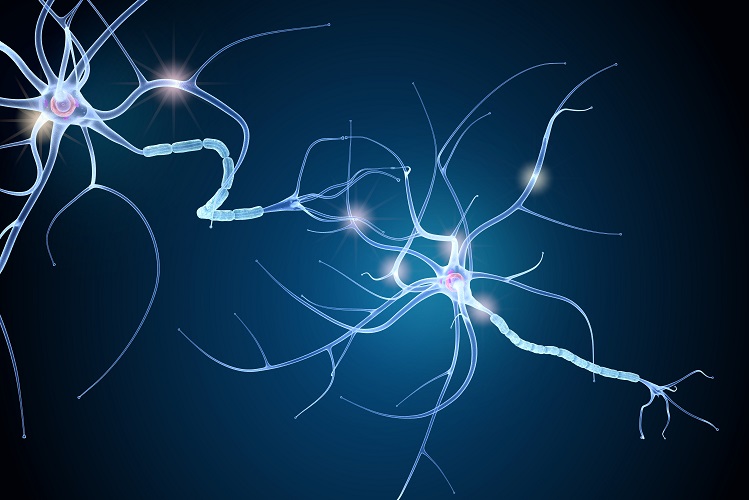
The terminal boutons or terminal buttons at the end of the terminals respond to the action potential by releasing neurotransmitters. These cross the synaptic cleft and activate the same process in a postsynaptic neuron. Alternatively, a few neurons send an ion-induced charge through small channels that cross into the postsynaptic membrane of the next cell. In chemical synapses, the action potential comes to an end at the terminal and must initiate at the axon hillock of the next nerve cell. In electrical synapses, the action potential simply continues until it reaches the last cell in the chain.
To initiate a neuron action potential, the membrane of the axon hillock must depolarize. The inside of the membrane is around -70mV when inactivated (resting potential). Depolarization means bringing this charge higher to more closely match the neutral charge at the outer side of the cell membrane. This is done by allowing large numbers of positively-charged sodium ions to flow into the cell. Depolarization will occur whether the incoming signals are weak or strong – even if the inside charge only reaches -69mV, this is still depolarization. This amount of depolarization is not, however, enough to cause an action potential.
The trigger for an action potential is a big enough signal from the dendrites – if the dendrite signal is weak, there will be no action potential. Only when depolarization brings the charge of the inner side of the cell membrane to at least -55mV can an action potential be initiated. There are no weak action potentials – they are all the same size; only their firing frequency changes. This is the ‘all or nothing’ principle of neuron action potentials.
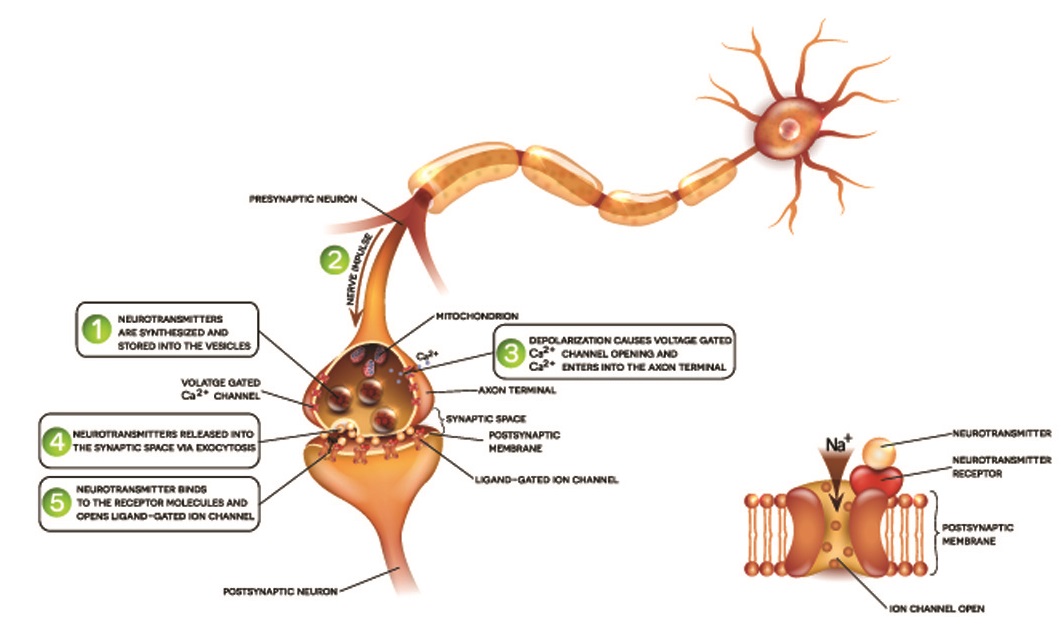
Once the action potential has been initiated, it must travel to the neuron terminals, usually along the axon. This requires the influx of sodium ions via channels that are activated by voltage changes. Once a channel has opened and allowed sodium ions to enter the cell, it must close for a short period. Because of this, the action potential only moves in one direction. Only a very limited number of neurons can send action potentials in two directions.
The more positive charge is pulled along the axon, attracted by the negative charge of the next section. As the positive charge moves forward, the section behind – with its sodium-ion channels temporarily closed off – allows positively-charged potassium ions to exit the cell and returns to -70mV, known as the resting potential of a neuron cell membrane. This process is called repolarization.
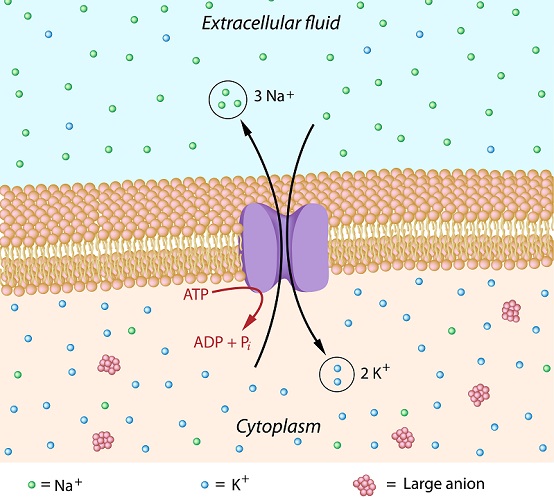
Hyperpolarization occurs because it takes the cell time to adjust. Until equilibrium is regained through natural ion diffusion along a concentration gradient, the membrane potential could be in the region of -80 mV for one or two nanoseconds. It is extra polarized – hyperpolarized. This does not last long and the resting potential of -70mV is reinstated.
Neuron Diseases
Neuron diseases affect motor, sensory, or sometimes both types of neuron. A motor neuron disorder will lead to muscle weakness or paralysis, while sensory neuron disorders can result in changes in the five senses and proprioception.
The most commonly-discussed diseases of neurons are multiple sclerosis, diabetic neuropathy, and motor neuron disease. The last of these has become globally recognized as the cause of death of the theoretical physicist Stephen Hawking. Motor neuron diseases will be looked at in more detail further on.
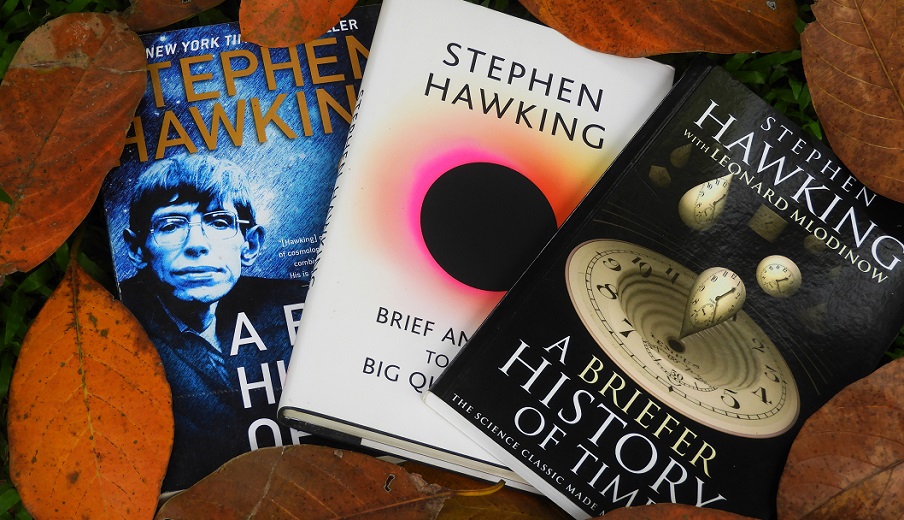
In multiple sclerosis, myelin damage affects action potential transmission along myelinated axons. As all of the major motor and sensory fibers have myelin sheaths, the effects can be broad – tingling sensations to pain, loss of vision, slower and less coordinated reflexes, and muscle and sphincter weakness. Myelin-producing Schwann cells can repair damage in several weeks, but auto-immune diseases are chronic disorders; unless treated, damage will progress. There are many other types of demyelinating disease but multiple sclerosis is by far the most widely known.
Sensory neuron diseases (SND) have various causes. Vitamin deficiencies (sometimes vitamin toxicity) have been well researched. For example, vitamin B deficiency is known to cause cognitive changes within the central nervous system, and vitamin E deficiency can affect the function of the cerebellum as well as the nerves of the digestive system.
Toxins in the form of heavy metals also cause damage to the nerves – arsenic poisoning causes the cell membrane potential to drop meaning there is less chance of an action potential being initiated. Arsenic also changes the form of certain proteins necessary for optimal nerve function such as calpain. Interestingly, arsenic poisoning also causes thiamine (vitamin B1) deficiency. These changes are predominant in sensory neurons.
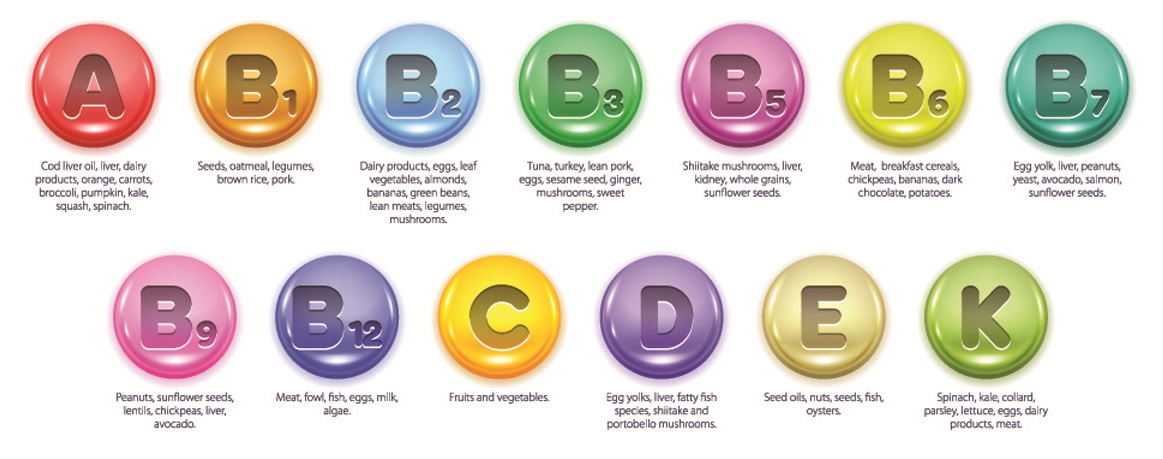
Cancerous tumors can grow and push on nerves, causing symptoms; chemotherapy directly damages the nerves and again, usually affects the sensory neuron. Chemotherapy-induced peripheral neuropathy (CPIN) causes pain, pins and needle sensations, and numbness. Certain types of cancer-fighting drugs seem to affect the function of ion channels.
Diabetic Neuropathy
Diabetic neuropathy or diabetic polyneuropathy (DPN) is the result of consistently high glucose levels in the blood and seems to directly affect the ganglions of the spine. Again, sensory neurons are usually affected. It is estimated that 50% of diabetics will experience some level of diabetic neuropathy during the course of their disease.
The main symptom is sensory loss, usually in the lower limbs. Someone with diabetic neuropathy may not feel a cut to the foot and later suffer from an infection. Motor nerves are also affected but at a much slower rate. Tests to diabetic skin samples show there are fewer sensory axons in the epidermis; one theory is that the soma of these sensory axons that sit in the ganglia slowly die off and the neuron terminal is the last to go.
Another reason why neurons become damaged is diabetic microvascular disorders where the presence of glucose changes the consistency of the blood. The capillaries also thicken in response. This means less oxygen and nutrients are transported to various tissues, including nerve tissue.
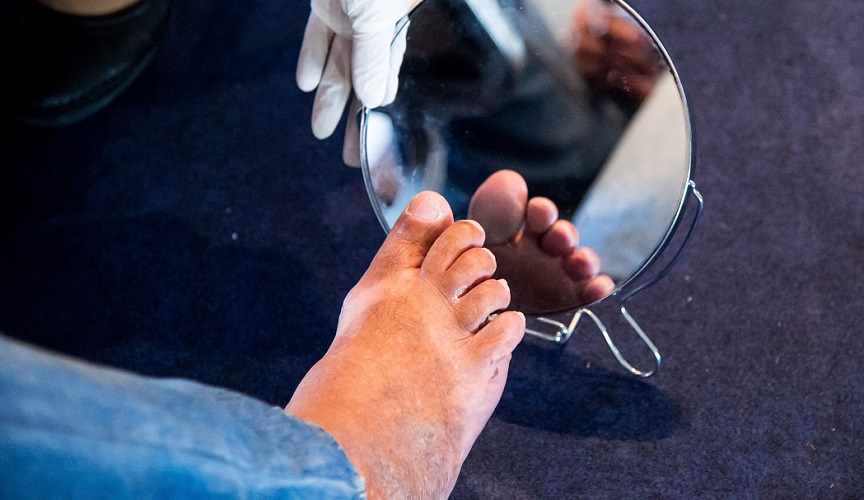
Diabetics are more likely to suffer from localized neurological disorders such as carpal tunnel syndrome and ulnar neuropathy at the elbow (cubital tunnel syndrome). The less common diabetic amyotrophy describes an associated motor neuron disorder that leads to muscle wasting and weakness, especially in the legs, and low-level reflex responses.
Motor Neuron Diseases
Motor neuron diseases (MNDs) are progressive neurological disorders that affect skeletal muscle activity. Problems with walking, speaking, swallowing, and even breathing can develop over time. Men are more likely to develop motor neuron disease and this group of diseases can affect approximately one man in every 100,000; around 3 women in every 100,000 are at risk. Often symptoms do not begin to occur until later on in life.
Most health departments provide fact sheets about motor neuron disease. Diseases can be inherited or develop during one’s lifetime. The upper motor neurons, lower motor neurons, or both can be affected. Upper motor neurons travel from the brain to the cranial nerves or spinal cord; lower motor neurons from the spinal cord to the muscles.
Sensory neurons are much less affected and an affected person will not experience cognitive changes. While the cause of ALS is not known, much research is being done as only 10% of sufferers survive longer than ten years after diagnosis. If you raised money during the Ice Bucket Challenge in the summer of 2014, you were supporting ALS research.
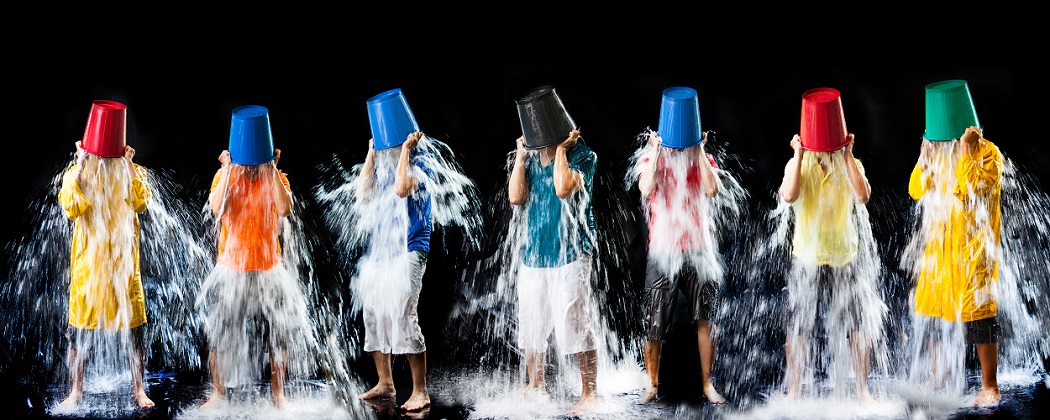
The most common form of motor neuron disease is amyotrophic lateral sclerosis (ALS) or Lou Gehrig’s disease. This disease tends to develop after the age of forty and the first symptoms are generalized muscle weakness and stiffness. As these symptoms are seen as age-related, they are often ignored.
It has been noted that some professional-level sports seem to be a risk factor, specifically those linked to repetitive head trauma such as football, soccer (‘heading’ the ball), and boxing.
Pediatric motor neuron disease occurs before the age of eighteen and is the result of an inherited gene mutation. Â Floppy baby syndrome or spinal muscular atrophy (SMA) is the leading genetic cause of death in children under two years.
When born, babies with SMA have trouble sucking, swallowing, and breathing. They do not cry when they are born. The mildest form of SMA is not immediately detectable but the child will be slow to walk and can only manage this activity for very short periods. These children nearly always require a wheelchair as they become older; the mild form of the disease is not fatal.
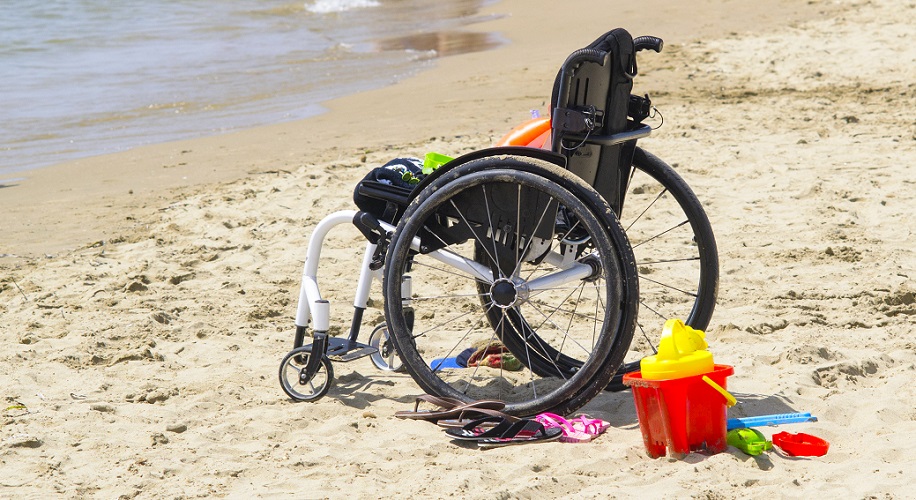
Artificial Neuron
An artificial neuron is a mathematically-based connection point in a (currently) artificial neural network. Computational neurobiologists use artificial neuron networks to simulate biological neuron circuits.
These simulated networks have led to the recent development of ‘bionic’ neurons at the University of Bath in the UK and in collaboration with scientists from Bristol (UK), Zurich (CH), and Auckland (NZ) Universities. To develop this microchip, they used artificial neuron networks to bypass the complex biological processes that make initial stages much more complicated. These tiny chips receive and process presynaptic messages and transmit them to postsynaptic cells in models. Research is now moving towards tests on living neurons.
It is hoped that artificial neurons will put an end to sensory and motor neuron diseases. The scope of artificial neurons is huge, and this new technology may cure a whole host of chronic neurological disorders.
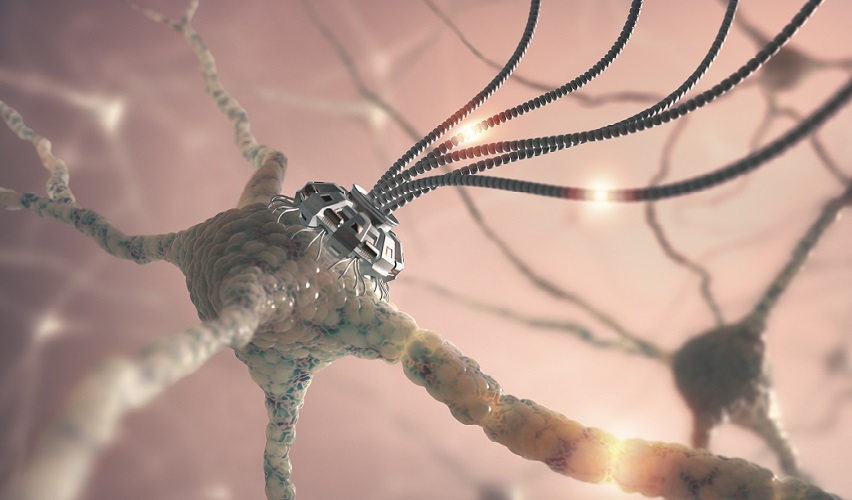
Quiz