Definition
While the capillary network used to be thought of as a permeable bridge between non-permeable arteries and veins, it is now known that these extensive structures help to regulate blood flow as well as water, gas, waste, and nutrient exchange. A capillary is a microvessel, as are its immediate neighbors the arterioles and venules; it is a fragile but essential part of the circulatory system.
Capillary Anatomy
Capillary anatomy is simple. Each capillary, traveling from lumen to outer wall, consists of:
- Endothelium (blue in below image)
- Basement membrane (pink)
- Surrounding connective tissue (light gray dots)
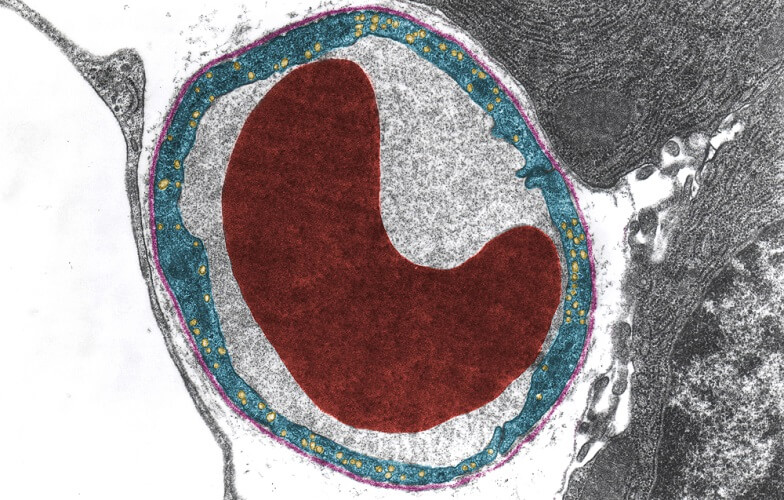
Capillaries cannot efficiently contract or relax as they have no muscle layer, although some contain contractile pericyte cells that allow a small degree of capillary wall adjustment.
Each capillary measures between five to ten micrometers in diameter. Researchers estimate the average human body contains around ten billion capillaries; if place end-to-end they would stretch for about 25,000 miles. About 5% of the total blood volume is found in the capillaries at any one time.
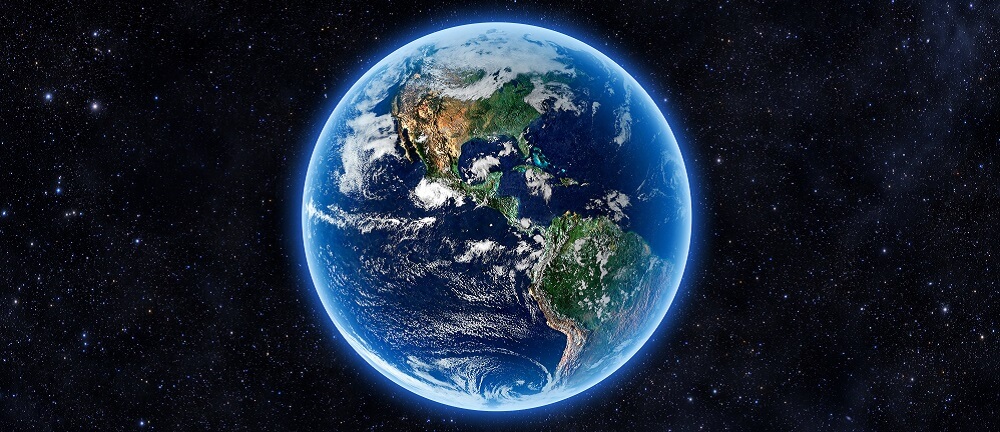
Capillaries are directly connected to arterioles, each other, or venules. Because the high blood pressure of larger arteries would damage the delicate capillaries, no capillary is directly attached to them.
Blood pressure is highest as blood is pumped from the heart into the aorta and pulmonary artery. This blood travels into smaller arteries and then into arterioles before arriving at a capillary network. Normal capillary pressure is between 10.5 and 22.5 mmHg, although some regions have much lower pressures.
Endothelium
The capillary endothelium is a single-celled layer that faces the lumen. All blood and lymph vessels have an endothelium composed of vascular endothelial cells (ECs); however, there are differences between their function and structure. We now know that new veins and arteries are formed from the capillary bed, with the capillary endothelium playing the role of a plastic layer with the ability to differentiate into venous and arterial endothelial layers.
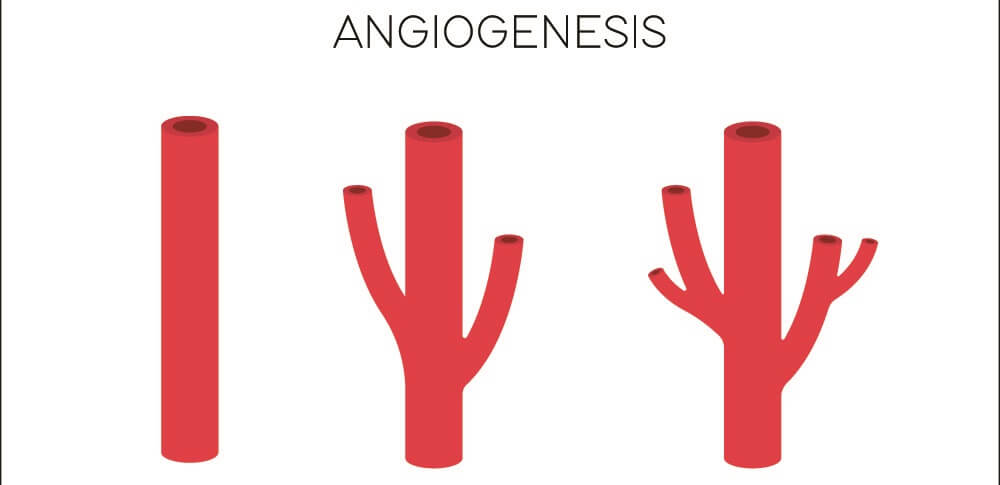
The endothelium is directly exposed to the blood and its many components. It is here that nutrients, oxygen, carbon dioxide, water, and waste products are filtrated or absorbed from or into the blood circulation. This means that specific capillary regions function differently – the capillaries in the kidney glomerulus, for example, are under a higher degree of pressure to enable more efficient solute exchange. Other capillary network locations – such as the spleen – serve as storage systems for large numbers of red and white blood cells and platelets.
In the last ten years, an integrated proteoglycan and glycoprotein network has been discovered that forms an absorbent mesh within the vascular endothelium layer. This is called the endothelial glycocalyx. Together, the glycocalyx and endothelium form the endothelial surface layer (ESL). Damage to the glycocalyx is an early sign of several chronic pathologies, including diabetes. We still know very little about this gel-like layer but its importance is rapidly gaining momentum as research associate glycocalyx damage with an extremely wide range of pathologies.
Thanks to the presence of pericytes placed around the endothelium cells, some capillaries can contract in a very limited way. Pericytes are best known for their role in the production of fibroblasts but, in the presence of calcium, can contract or relax to help to adjust the capillary diameter.
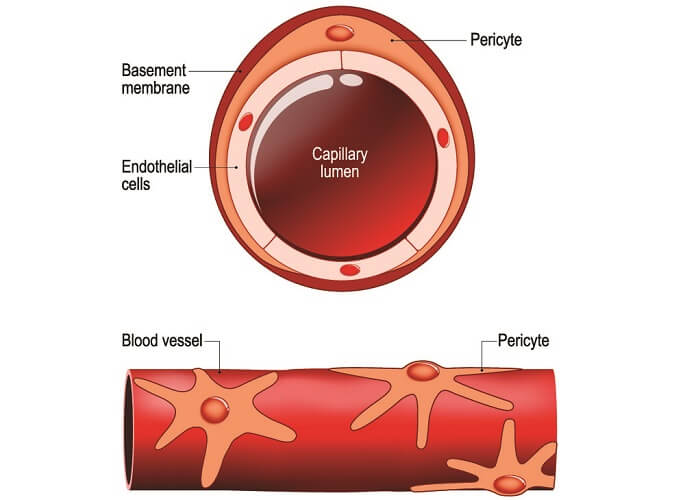
Endothelial cells also contain small indentations called caveolae (caves) that help molecules pass through the membrane by way of transcytosis. More will be said about transcytosis further on. Where endothelial cells join, only very specific molecules can pass through.
Basement Membrane
Endothelial cells sit on a basement membrane that, in healthy subjects, is a regular, thin layer. The basement membrane contains the components necessary for cell differentiation (when creating new arterioles and venules, for example), acts as a further barrier to aid or hinder gas, nutrient, and waste exchange, and is an adhesive layer to which endothelial cells and pericytes attach.
Connective Tissue
The collagenous tunica adventitia is composed of loose connective tissue and certain cells that include macrophages, mast cells, and fibroblasts. This layer helps a capillary to keep its shape and provides a limited level of protection for the ESL. It also attaches the vessel to neighboring structures. It is often not included in capillary structure diagrams.
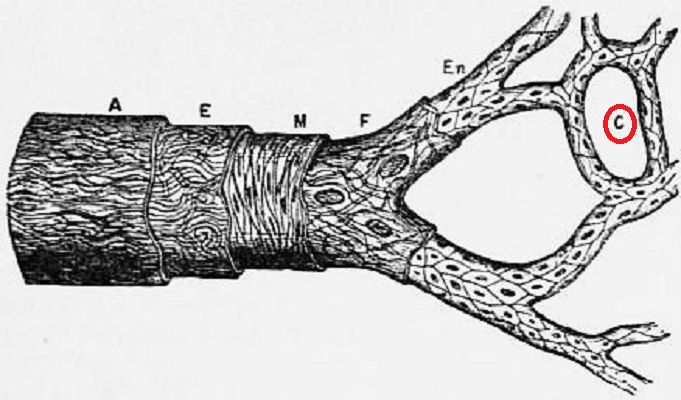
Capillary Types
There are three main types of capillary: continuous, fenestrated, and discontinuous.
Continuous endothelium is permeable but only to a degree. Any exchange occurs through the endothelial cell that either wraps around itself to make a lumen as a single-celled layer or joins to another endothelial cell at a tight junction that does not allow larger molecules to pass through. Only small water-soluble molecules can get through this gap. Continuous capillaries are most commonly found in muscle, lung, skin, and connective tissues.
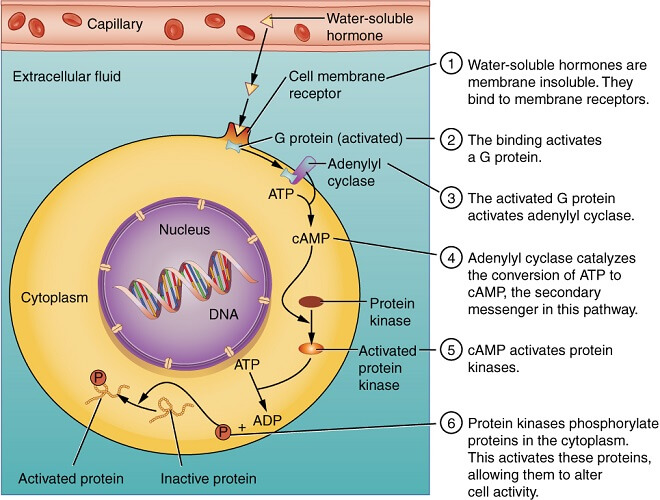
Where more than one cell forms a capillary wall and these cells are not joined via almost impenetrable seams, the gaps between them create windows – fenestrae. The basement membrane remains intact but molecules can pass through the endothelial pores. This provides a higher degree of permeability. Fenestrated capillaries are most often found in kidney glomeruli, the peritubular capillary beds surrounding nephrons (below image), endocrine glands, intestinal villi, and the pancreas.
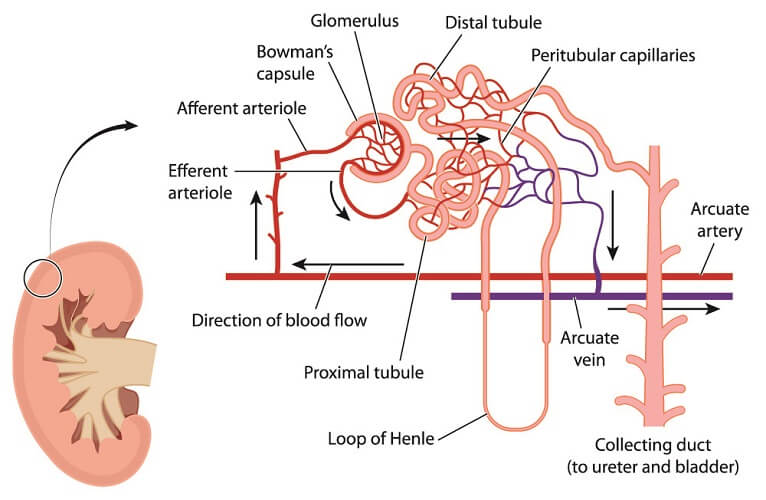
The highest degree of permeability is found in discontinuous capillaries. Not only are there gaps between endothelial cells, the basement membrane is also discontinuous. Unlike fenestrated capillaries where larger (macro) molecules cannot filter through the intact basement membrane, discontinuous capillaries allow much larger molecules to pass through. This is why you are most likely to find this capillary type in the red bone marrow that produces new (large) blood cells, and in the lymphoid organs and liver.
Sinusoid capillaries are found in the liver, spleen, lymph nodes, some endocrine glands, and the bone marrow. They can be made of any of the above endothelium types. Below, you can see a sinusoid capillary in the liver.
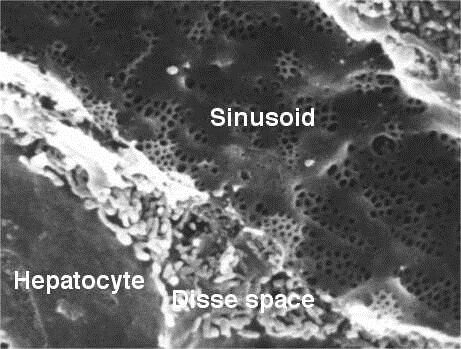
Capillary Function
Capillary function is primarily the transport of blood components into surrounding tissues and the transport of waste products, cells, enzymes, factors, and hormones from tissue to bloodstream. As we have already seen, some molecules can pass through endothelial cell junctions. In the case of continuous capillaries, pores between endothelial cells will only allow the smallest water-soluble molecules to pass through. Others must pass through the endothelium via the endothelial cell.
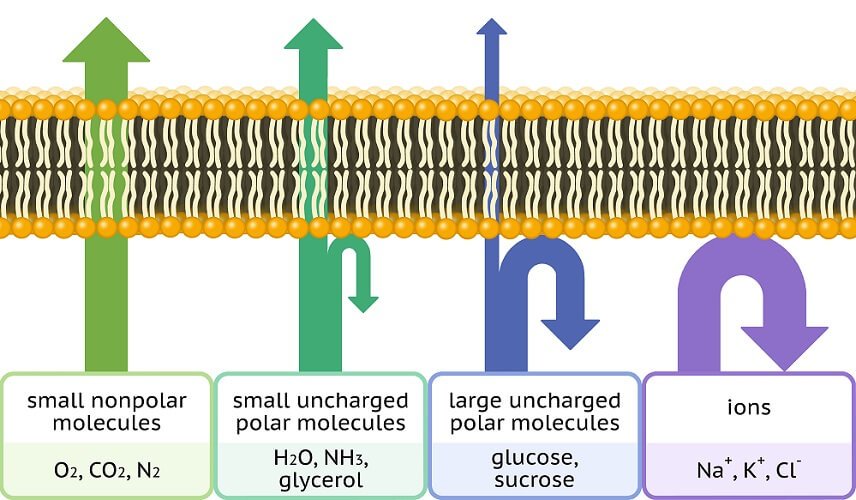
Lipid-soluble molecules can pass through the phospholipid endothelial cell membrane and so enter (and exit) the cell without using precious cellular energy. Water-soluble molecules can pass through the gaps between cells, also without the use of energy. In the case of larger molecules, a less efficient method is required – transcytosis.
Transcytosis requires energy and involves selective transport channels across the endothelial cell membrane (endocytosis), transport across the cell membrane within vesicles (vesicular transport), and exocytosis – the dissolving of a vesicular membrane into the cell membrane and out of  the cell on the opposite side.
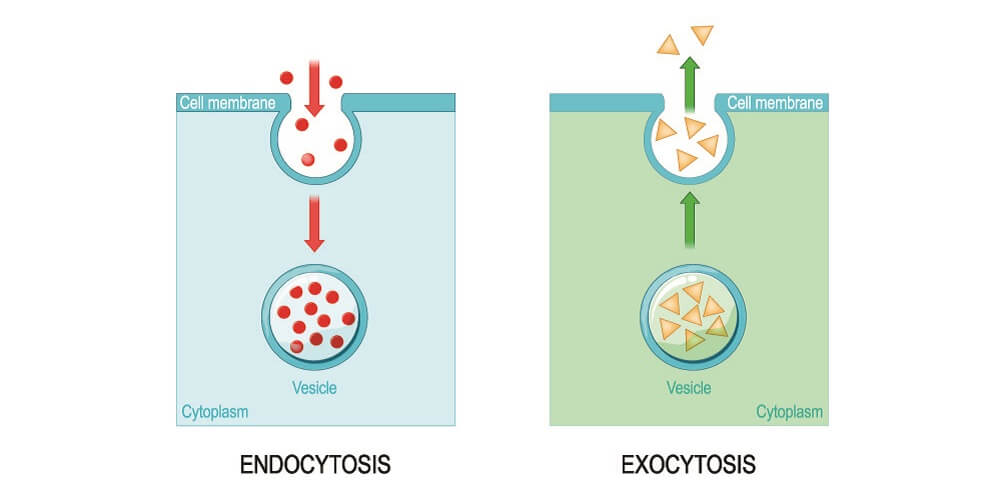
What passes through the cell and what passes through the gaps between depends on the solubility of a molecule. Lipid-soluble substances that include oxygen, carbon dioxide, some hormones, and fatty acids travel through the cell via simple, low-energy diffusion. Larger proteins must use transcytosis. Water-soluble molecules that include ions, glucose, and amino acids are transported through the gaps between capillary endothelial cells.
The mechanism of low-energy exchange relies on Starling forces. Starling forces in this case describe the difference between hydrostatic and oncotic pressures between a capillary and its surrounding tissue. Hydrostatic pressure is the pressure caused by the fluid (blood) against the endothelium of the capillary – specifically called the capillary hydrostatic pressure (CHP). Higher levels of hydrostatic pressure push water molecules – with or without dissolved components – out of the capillary and into the surrounding tissue via a mechanism called filtration. As this fluid enters the tissues, tissue hydrostatic pressure – interstitial fluid hydrostatic pressure (IFHP) rises. To ensure capillary exchange does not become limited and cause edema (tissue swelling), lymph vessels in the surrounding tissues constantly absorb excess fluid.
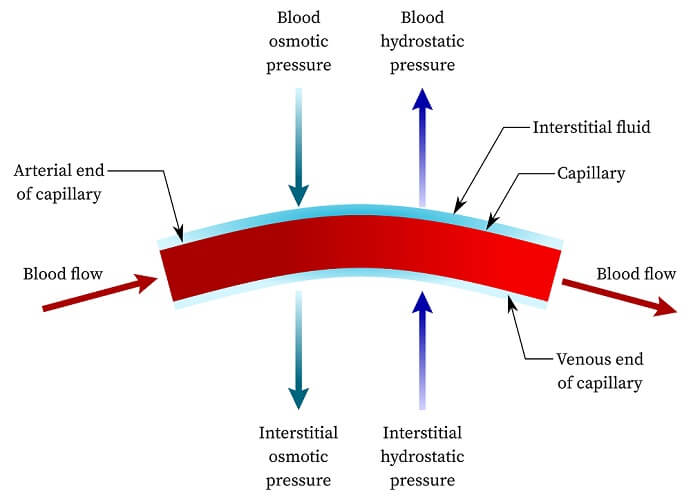
Oncotic pressure relates to the presence of plasma proteins – most commonly albumin – in the interstitial spaces outside the capillary. These cause reabsorption and the movement of water-soluble waste and water back into the capillaries from the surrounding tissues. Where the concentration of water molecules is lower than the other side of a semi-permeable membrane due to higher levels of proteins, the higher solute concentration will pull water back into the capillary. This drawing in of water makes the CHP rise.
Both filtration and reabsorption regulate the rate of exchange through osmosis and reverse osmosis using plasma proteins as a solute.
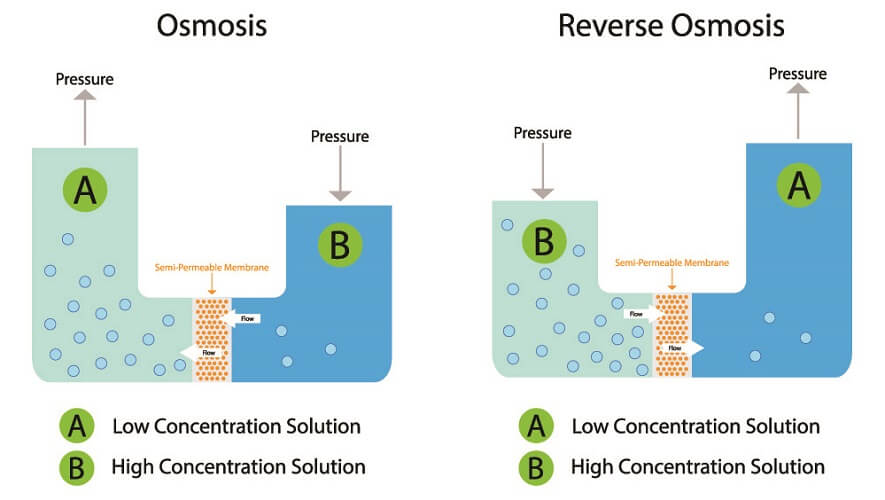
While it takes longer for a lipid-soluble or large molecule to pass through an endothelial cell, this process occurs very frequently. Only approximately 1% of the capillary epithelium contains gaps or pores for water-soluble molecular transport. Furthermore, what can and cannot pass through a capillary network depends on its location within the body.
Capillary Damage
Systemic capillary damage can produce serious consequences while localized injuries tend to have little effect. Broken capillaries on face and nose, for example, are simply overly-dilated surface capillaries that cause unsightly red lines. They are only treated for cosmetic purposes.
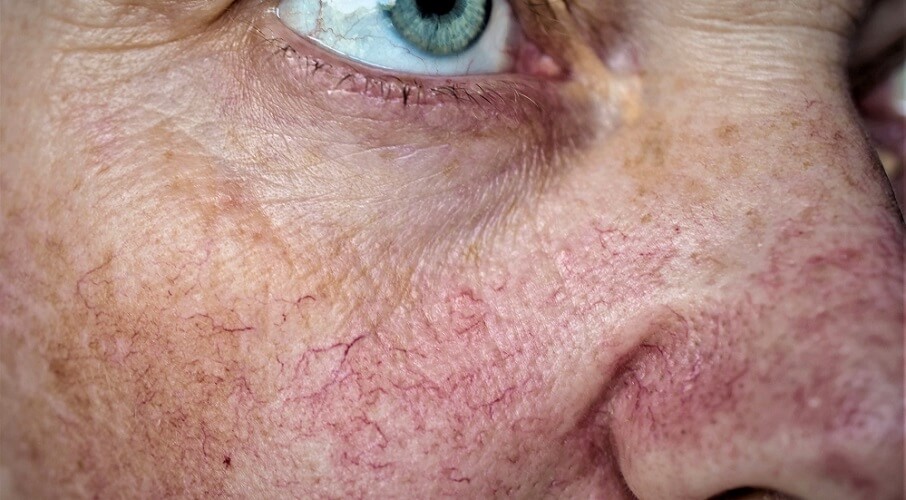
Broken capillaries – usually the result of a knock – lead to localized blood loss until the clotting mechanism initiates. These are often superficial injuries; where a capillary bleed occurs defines the level of damage. A small bruise on the arm will fade; vitreous capillary bleeding in the eye, however, may cause loss of vision – the blood blocks any light from traveling through the eye. This is usually short-term and rectifies once new capillaries have formed to replace the broken ones.
Capillary leak syndrome, on the other hand, disturbs the Starling forces and causes edema – high levels of interstitial fluids and swelling. This can become fatal without treatment and seems to be due to damage to the endothelium and glycocalyx, allowing unregulated molecules and fluid to pass into the surrounding tissue.
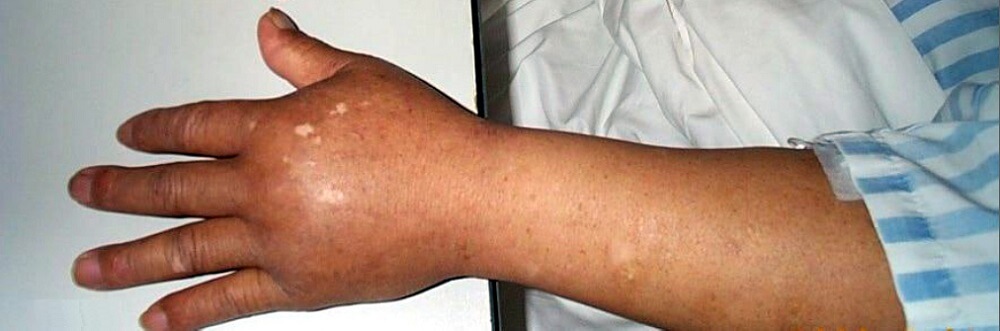
In diabetics, increased capillary permeability on a lower scale than capillary leak syndrome may be linked to the exposure of the glycocalyx to permanently-high blood glucose levels. As insulin and glucose must at some point pass through the capillaries, any damage to this component of the ESL would increase glucose dysregulation inside the body. Diabetics are also prone to lower capillary perfusion of the extremities, meaning the amputation of toes is sometimes necessary. A systemic disease like diabetes has countless negative effects on the body; these are not only limited to the capillaries. However, many subsequent symptoms are the result of lower oxygen and nutrient supply to the tissues caused by long-term blood vessel damage.
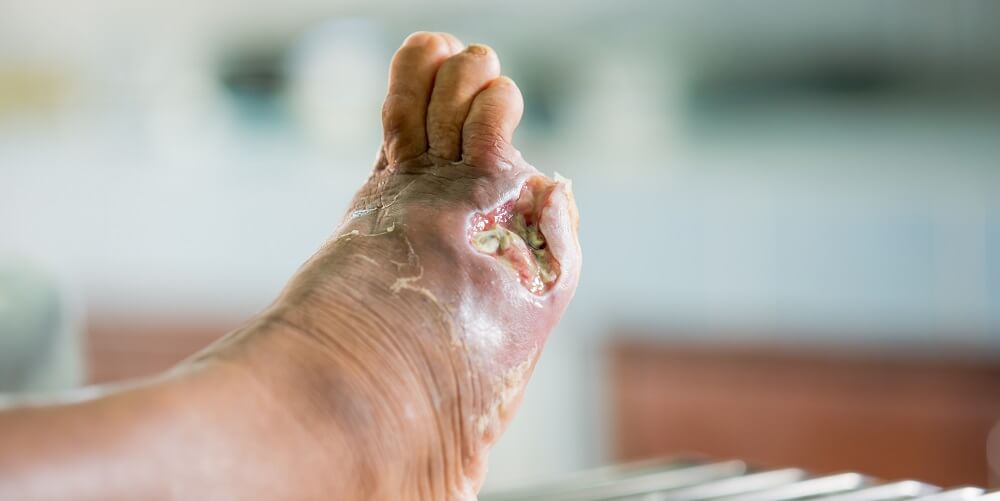