AP Biology 3.5 - Photosynthesis
This section of the AP Biology curriculum covers photosynthesis and the reactions that make harvesting energy from the sun possible. We’ll start by going 2.3 billion years into the past to analyze the origins of photosynthesis. Then, we’ll go over a quick overview of the entire process. Finally, we’ll dive into the complexities of the light-dependent reactions that take place in the thylakoid membrane (including the photosystems and electron transport chain) and see how the products of these reactions feed the light-independent reactions of the Calvin cycle that ultimately create glucose. We’ll see not only how the photosystems are able to harvest light energy using pigments like chlorophyll, but we will also see how this energy is passed to the Calvin cycle using ATP and NADPH!
Video Tutorial
The following video summarizes the most important aspects of this topic!
To watch more tutorial videos like this, please click here to see our full Youtube Channel!
Resources for this Standard
For Students & Teachers
- Overview & Video Tutorial (This article)
- Quick Test Prep
- Crossword Puzzle
For Teachers Only
ENDURING UNDERSTANDING
ENE-1
The highly complex organization of living systems requires constant input of energy and the exchange of macromolecules.
LEARNING OBJECTIVE
ENE-1.I
Describe the photosynthetic processes that allow organisms to capture and store energy.
ENE-1.J
Explain how cells capture energy from light and transfer it to biological molecules for storage and use.
ESSENTIAL KNOWLEDGE
ENE-1.I.1
Organisms capture and store energy for use in biological processes–
- Photosynthesis captures energy from the sun and produces sugars.
- Photosynthesis first evolved in prokaryotic organisms.
- Scientific evidence supports the claim that prokaryotic (cyanobacterial) photosynthesis was responsible for the production of an oxygenated atmosphere.
- Prokaryotic photosynthetic pathways were the foundation of eukaryotic photosynthesis.
ENE-1.I.2
The light-dependent reactions of photosynthesis in eukaryotes involve a series of coordinated reaction pathways that capture energy present in light to yield ATP and NADPH, which power the production of organic molecules.
ENE-1.J.1
During photosynthesis, chlorophylls absorb energy from light, boosting electrons to a higher energy level in photosystems I and II.
ENE-1.J.2
Photosystems I and II are embedded in the internal membranes of chloroplasts and are connected by the transfer of higher energy electrons through an electron transport chain (ETC).
ENE-1.J.3
When electrons are transferred between molecules in a sequence of reactions as they pass through the ETC, an electrochemical gradient of protons (hydrogen ions) is established across the internal membrane.
ENE-1.J.4
The formation of the proton gradient is linked to the synthesis of ATP from ADP and inorganic phosphate via ATP synthase.
ENE-1.J.5
The energy captured in the light reactions and transferred to ATP and NADPH powers the production of carbohydrates from carbon dioxide in the Calvin cycle, which occurs in the stroma of the chloroplast.
EXCLUSION STATEMENT
Memorization of the steps in the Calvin cycle, the structure of the molecules, and the names of enzymes (with the exception of ATP synthase) are beyond the scope of the course and the AP Exam.
3.5 Photosynthesis Overview
Billions of years ago, humans could not survive on Earth – even if we had a time machine to get there. Back then, there was literally no oxygen in the atmosphere. It was not until about 2.3 billion years ago that the process of photosynthesis evolved in primitive cyanobacteria. With the evolution of this process, the Earth’s atmosphere underwent a massive shift. As the cyanobacteria reproduced and filled the Earth’s oceans, massive amounts of oxygen spilled into the atmosphere. This forever changed the course of evolutionary history, leading to the complex forms of life that we see today.
The process of photosynthesis captures energy from the sun to produce glucose. This process not only changed the world to allow for all the forms currently extant, but the process literally captures enough energy from the sun to feed ecosystems all over the world. But, how does this process work? How can simple cellular components be more efficient than a solar panel? What is the difference between light-dependent and light-independent reactions? These questions will definitely be incorporated into the AP Test. So, stick with us as we cover everything you need to know about photosynthesis!
Around 3 billion years ago, the Earth was water, rock, and a very simple atmosphere made of mostly nitrogen and carbon dioxide. If there was any oxygen, it was certainly not enough to sustain modern organisms. Early cells formed in the primordial soup of the ocean and some of these cells evolved the ability to photosynthesize. These organisms were the earliest version of cyanobacteria. As these cells multiplied and spread throughout the world’s oceans, the massive amount of photosynthesis taking place added huge amounts of oxygen to the Earth’s early atmosphere! By analyzing rocks, fossils, and other sources of ancient information, scientists estimate that the early atmosphere became highly oxygenated around 2.3 billion years ago.
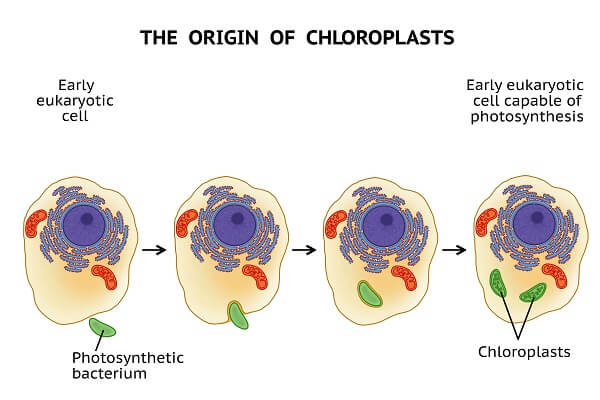
This gaseous oxygen, which is an essential part of the process of aerobic respiration, likely gave rise to other types of bacteria that could utilize the energy being captured by these early cyanobacteria. This could have led to the process of endosymbiosis – covered in section 2.11 – that created the first eukaryotic organisms around 2 billion years ago. After nearly a billion more years of evolving in the oceans, early eukaryotic algae evolved into the first land-dwelling plants that were likely similar to ferns. It was only after several million more years that flowering plants emerged and helped animals expand into the vast diversity we see today!
As for the evolution of the macromolecules that allow for photosynthesis, we know a little less about how these molecules came to be. These molecules – such as chlorophyll – have a structure that allows the energy to be extracted from passing photons to collect energy from the sun. There are several pigment molecules that can collect energy from photons, but only chlorophyll-a is present in cyanobacteria, algae, and vascular plants. Therefore, it is assumed that this was the first photosynthetic pigment that arose in ancient bacterial populations.
Think about this… while photosynthesis may seem like an abstract concept as we start to go through the individual processes that make it possible, photosynthesis is literally feeding the world. Whether it is an herbivore feeding off of the excess energy that plants have accumulated or a carnivore feeding off of the energy that herbivores have gathered from plants, most life on Earth depends on the process of photosynthesis to gather energy from the sun and store it in the bonds of organic molecules. So, as we start to learn about how photosynthesis works at a molecular level, keep in mind that the energy you need to survive is literally being generated by this process.
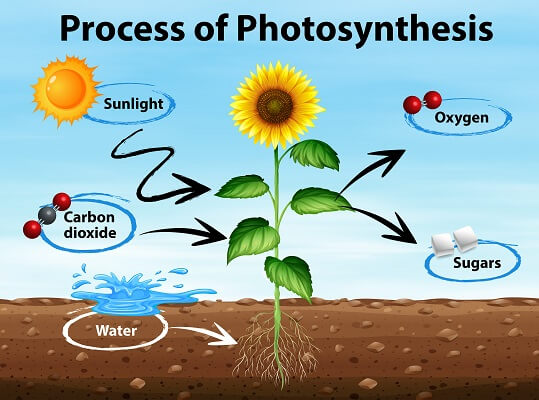
Before we dive into the different reactions that make photosynthesis possible, let’s go over a quick overview of the entire process. In its essence, photosynthesis is a process that takes water, carbon dioxide, and the energy from sunlight to create glucose molecules while releasing oxygen as a byproduct. While the overall reaction is simple enough to understand and you can literally count all the atoms involved to check that they balance, the actual process of photosynthesis requires dozens of individual reactions and enzymes that work sequentially to slowly recombine the carbon atoms from carbon dioxide into much larger glucose molecules.
These many reactions can be broken into two sets of processes: the light-dependent reactions and the light-independent reactions. These two sets take place in different parts of the chloroplast. The light-dependent reactions happen right on the thylakoid membranes in the stacks of grana, while the light-independent reactions happen in the stroma (or space between the grana) within each chloroplast. As we will see in the following slides, the light-dependent reactions are responsible for extracting energy from the sun and storing that energy in the bonds of ATP molecules and electron carriers like NADPH. This process uses water and releases oxygen. The light-independent reactions use the energy from ATP and electron carriers to combine carbon dioxide molecules into glucose molecules.
The light-dependent reactions of photosynthesis take place on thylakoid membranes, which house a number of important enzymes and pigments that make the process of extracting energy from light possible. Let’s take a closer look!
If we take a closer look at the stacks of grana within a chloroplast, we can see that they are simply a complex stack of thylakoids. Each thylakoid is simply a chamber created by this folded membrane. But, we have to zoom in even further to get to the proteins embedded in the thylakoid membrane that make photosynthesis possible: the photosystems.
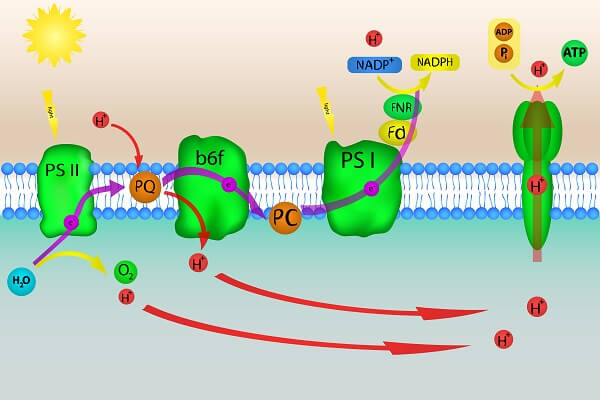
The photosystems, including photosystem I and photosystem II, are integral membrane proteins embedded directly into the thylakoid membrane. Keep in mind that these photosystems were named in the order that they were discovered, but they form an electron transport chain that starts with photosystem II. This process starts with photons hitting photosystem II. This enzyme houses chlorophyll pigment molecules, which trap the energy from photons using a magnesium atom in the center of the structure. This energy is passed through photosystem II, which catalyzes a reaction that breaks a water molecule apart into oxygen atoms and hydrogen ions.
The electrons from this reaction start flowing through the electron transport chain, while the hydrogen atoms start accumulating in the thylakoid lumen. The electrons travel through a molecule of plastoquinol (or simply PQ) before they reach a protein proton pump known as cytochrome b6f. This proton pump uses energy from the electrons to pump more hydrogen ions into the thylakoid membrane. The electrons then make their way through another molecule known as plastocyanin (or PC), before reaching photosystem I. Photosystem I is another pigment-containing enzyme. Instead of splitting water, photosystem I uses the energy it gathers to re-energize the electrons. The re-energized electrons can then be added to the electron carrier molecule NADP to create NADPH. As a final step, the hydrogen ion gradient that has been established by this electron transport chain is passed through ATP synthase to produce ATP molecules.
The light-independent reactions are how chloroplasts utilize the energy captured in the light-dependent reactions to generate glucose. These reactions take place in the stroma of the chloroplast. The most significant of these reactions are concentrated within a process known as the Calvin cycle.
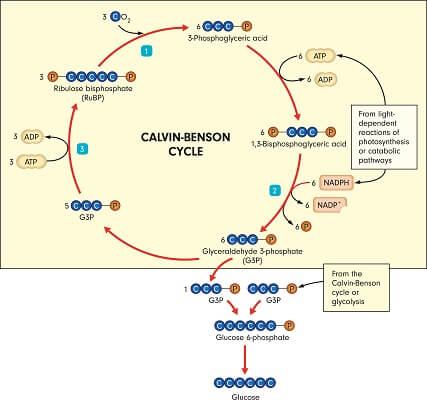
The Calvin cycle starts when an enzyme merges the end product of the Calvin cycle – ribulose biphosphate – with a carbon dioxide molecule. This process is known as carbon fixation because it “fixes” 1 carbon atom from carbon dioxide with an already-established 5-carbon chain. This creates a 6-carbon molecule that quickly breaks in half to form a 3-carbon molecule known as 3-phosphoglyceric acid. Using the ATP and NADPH from the light-dependent reactions, the Calvin cycle then rearranges this molecule into glyceraldehyde 3-phosphate (also known as G3P). This part of the Calvin cycle is called “reduction”.
This G3P molecule is essentially what you get when you break glucose molecules in half, and we will see it again when we start looking at the processes of glycolysis and aerobic respiration in the next section. A small portion of the G3P is exported from the Calvin cycle to produce glucose, while the rest of it remains in the cycle. The remaining G3P molecules will be used to make more ribulose bisphosphate in the final stage of the Calvin cycle known as “regeneration.” This allows the cycle to continue, starting back over at the carbon fixation stage.
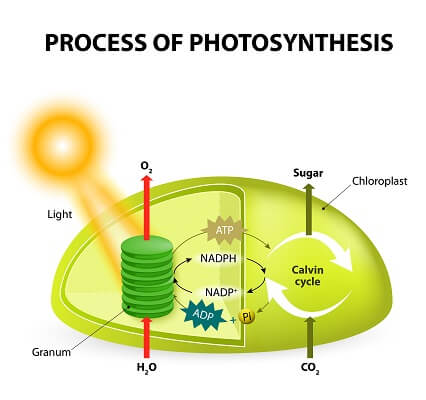
While there are some variations on how plants complete different aspects of this process, the basics of the Calvin cycle and carbon fixation remain the same in all photosynthesizing organisms and are responsible for storing a majority of the energy utilized on planet Earth!