[LS4-2] Factors Influencing Evolution
This standard focuses on how evolution takes place within a population, focusing specifically on the 4 key drivers of evolution: reproduction, genetic variation, competition, and survival.
Resources for this Standard:
Here’s the Actual Standard:
Construct an explanation based on evidence that the process of evolution primarily results from four factors: (1) the potential for a species to increase in number, (2) the heritable genetic variation of individuals in a species due to mutation and sexual reproduction, (3) competition for limited resources, and (4) the proliferation of those organisms that are better able to survive and reproduce in the environment.
Standard Breakdown
This standard has 4 specific parts that we will look at in detail:
Exponential Growth
Most species are capable of exponential growth. Think of a simple bacteria, dividing regularly every 30 minutes. In 1 hour, 1 bacteria becomes 4. At 2 hours, the population has grown to 16. By 24 hours, the population has grown to well over 200 Billion! In bacteria, this situation is easy to model mathematically:
2(number of generations) = total population
So, 24 hours at 1 generation every 0.5 hours, there are roughly 48 bacterial generations that could take place. In mathematical terms:
248 = 281,474,976,710,656 bacteria
If each bacteria weighs roughly 1 x 10-12 g, we can easily calculate the weight of this theoretical colony.
281,474,976,656 bacteria x (1×10-12g) = 281.474 g
That’s a lot of bacteria! Essentially, without any limits, one bacteria would grow to the same mass as a fat hamster in only 24 hours. Given enough time, theoretical growth would allow this bacteria to weigh more than the Earth itself!
This basic premise extends to all species that reproduce, as a single individual can create many offspring in a lifetime. However, there are many limits to growth, as we will see, which stop populations from growing beyond certain levels and lead to evolution!
Genetic Variation
To understand why the following events lead to evolution, it’s important to understand that there are differences between individuals within a population. While we consider the population a single unit because the organisms within the population can interbreed, each individual has slight differences in the genetic code.
These differences aren’t enough to create a different species, they just slightly change the function of some proteins and cellular reactions within the organism. This leads to observable variations within a population – such as fur color, metabolism efficiency, size, reproductive rate, and many other traits with slight variations.
As we will see, these genetic variations within the population become the basis for evolution. Some mutations are beneficial to specific individuals, allowing them to survive and reproduce more efficiently. Other variations are called deleterious mutations – these mutations do not help organisms survive or reproduce.
Competition for Limited Resources
The main cause of evolution is simple – competition for limited resources. Whether the organism is a plant, animal, fungi, or single-celled organisms, all organisms need resources like water, nutrients, oxygen, and sugar. The Earth – as a planet floating in the emptiness of space – has a limited amount of all of these resources.
As exponential growth rapidly expands a population in a given area, any resource can become a limiting resource – one that stops or slows population growth. For example, sunlight is a limiting resource for plants. Only so many plants can grow in a certain space before all the light is used up. But, plants may have other limiting resources as well – such as nutrients in the soil, carbon dioxide in the air, or available water.
In a closed system, such as a petri dish full of bacteria, the effect of competition for limited resources becomes clear. The bacterial population explodes as it finds a fresh plate of sugar-rich agar. The exponential growth continues until the sugar begins to run out. Without a source of energy, the bacteria go into a low-growth “stationary phase” where the population stalls out. Then, if no new nutrients are added and all the sugar is used up, the bacteria rapidly begin to die off, as seen in the graph below:
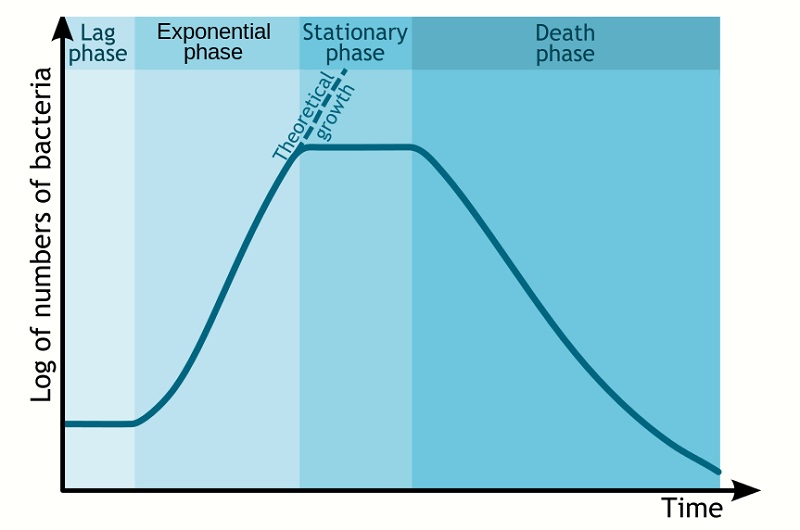
When resources are cycled and recycled within an ecosystem (as most matter is), this leads to a slightly different situation. Instead of a massive die-off, many populations tend to stabilize at their carrying capacity. This does not mean that competition for limited resources stops; it simply means that the reproductive success of these populations is limited to the nutrients each organism can compete for.
This, ultimately, leads to evolution.
Survival
The key to natural selection is survival. Organisms that survive can reproduce, and only reproducing organisms can pass on their genes. This ensures that only beneficial mutations get copied, leading to a change in the genes within a population. Single genes can control an entire observable trait, but most of the time many genes contribute to the phenotype (or visible trait). Through mutation and other mechanisms that increase variation in a population, new phenotypes evolve as new mutations are constantly tested in the environment.
Adapt, Migrate, or Die!
Essentially, in the face of competition for limited resources and with genetic variations that make each organism slightly different, each population faces three options.
First, the population can adapt to the environment through the process of natural selection. Because there is constant competition for resources, animals with beneficial mutations are more likely to survive and reproduce. This leads to the beneficial mutations becoming more prevalent in a population, and can even become “fixed” in a population (all individuals have the mutation) if they are beneficial enough to outcompete other mutations.
Second, populations can migrate into new environments. While they may not be well-adapted to these environments, they may find a new niche where there are plenty of resources to exploit. A great example of this is the migration of finches in the Galapagos Islands – some of which form entirely new species as they reach a new island and find a new niche to exploit. Migration may be one of the most efficient forms of speciation because natural selection in a new environment provides many new selective pressures that shape the incoming population into a new species.
Lastly, some populations cannot cope with the rate of change in an environment and thus become extinct. Extinction events have punctuated the fossil record, likely caused my massive environmental changes that decrease the availability of resources in certain areas. For a small population, such as an ant colony, this could be as simple as spraying a weed killer near the colony – exhausting their food resources and forcing them to migrate or die. On a global scale, massive changes in atmosphere composition, volcanic eruptions, and meteorite impacts all have the ability to massively change the resources available. In turn, this leads to the extinction of many species, typically followed by a period of rapid evolution as species compete to fill new niches in the new environmental conditions.
A little clarification:
The standard contains this clarification statement:
Emphasis is on using evidence to explain the influence each of the four factors has on number of organisms, behaviors, morphology, or physiology in terms of ability to compete for limited resources and subsequent survival of individuals and adaptation of species. Examples of evidence could include mathematical models such as simple distribution graphs and proportional reasoning.
Let’s look at this clarification a little closer:
Influence on Number Organisms
The number of organisms in a population or ecosystem is reliant on the DNA each organism carries. The DNA ultimately dictates how an organism can reproduce. A good way to show this is to discuss “K-” vs “r-” selected organisms. This r/K selection theory essentially shows that r-selected organisms reproduce in large numbers in order to maintain their population, whereas K-selected organisms usually invest more energy into a smaller number of offspring.
For example, this ladybug is an r-selected species. It produces many offspring, exhibits no parental care, and maintains or grows its population simply through sheer numbers of reproduction.
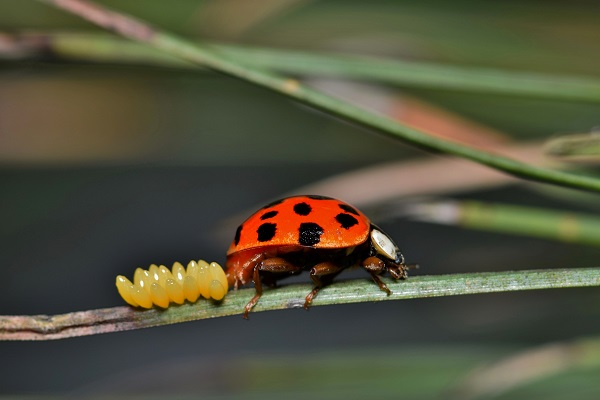
By contrast, elephants are a K-selected species. Female elephants typically give birth to one offspring at a time, raise and teach that offspring the skills it needs to survive, and typically do not give birth again until the first offspring is old enough to protect and defend itself. Instead of sheer numbers, elephants rely on careful parenting and a social community to ensure the offspring of most offspring. While this is a more energy-expensive method, it can also lead to increases in a population and can be more protected against temporary shocks to the ecosystem. (Humans are also K-selected.)
One good mathematical way to visualize these two different strategies is the “survivorship curve.” A survivorship curve plots how many offspring in a population survive compared to the total lifespan of the organism.
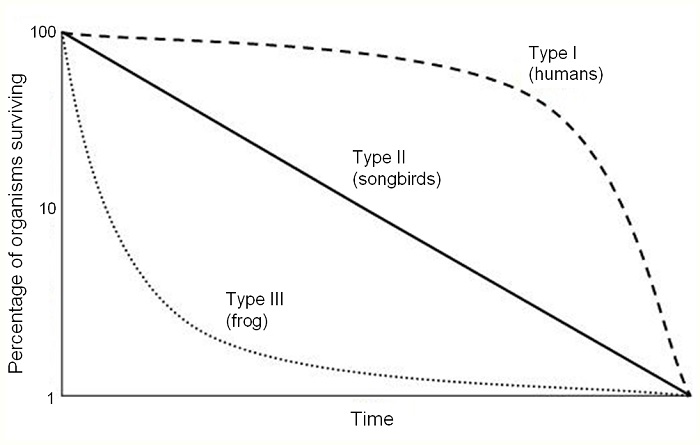
Type I (K-selected) organisms typically have very high survivorship while they are young. They are protected by their parents, fed regularly, and often are given times to develop larger brains. This leads to much higher survivorship within the offspring. By contrast, Type III (r-selected) organisms die in huge numbers when they are young. Many baby fish, amphibians, reptiles, and insects serve as food sources for a number of organisms. However, many of these creatures become more resistant to predators as they grow – leading to the relatively small number of adults that are able to survive and reproduce.
Influence on Behaviors
While behavior is still being actively explored by researchers, it is clear that most behaviors have at least some genetic component. These behaviors are typically called ‘innate’ behaviors because they are present from birth (or hatching). Innate behaviors, as such, are highly governed by genetics. As such, innate behaviors are “phenotypes” that can be observed and measured over time.
Learned behavior, by contrast, is often passed from the parental generation to the offspring. Elephants teach their young to dig up water sources, tigers teach their cubs how to effectively hunt, and whales have developed different “dialects” of whale calls between different pods. These behaviors may also be affected by genetics, though there is a much larger social and environmental component.
Influence on Morphology
Morphology is much more clearly dependent on genetic variation. Genes control proteins, which determine the expression of proteins, which determine the shape and content of cells, which ultimately form full organisms. Even minor mutations in the genes of an organism can produce variability within the population. By measuring populations over time, we can see this change in features by looking at a distribution of features on a graph. In fact, there are three different types of changes we can see: (1) Directional Selection (2) Stabilizing selection, and (3) Disruptive selection.
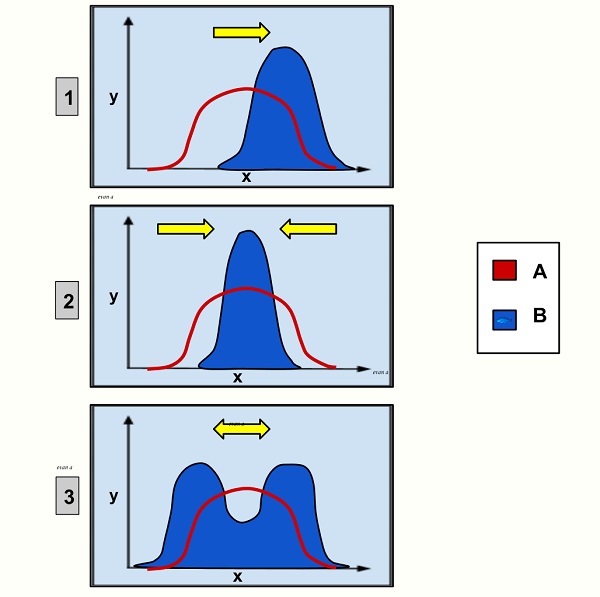
When an organism has a mutation in the DNA, the protein produced may not function, may function faster, or may function slower. The rate of how fast a specific protein functions (or if it functions at all) ultimately determines certain features and functions of cells. When this rate changes, certain morphological traits – such as height, number of teeth, bone strength, etc. – can change as well. Certain mutations may be beneficial, leading to the adaptation of morphology within a population (such as the expansion of feathers in dinosaurs after the impact of a globally-reshaping meteorite).
Another good example is seen in the fossil evidence showing whale evolution. The ancestors of whales and dolphins had 4 limbs, complete with feet and toes for walking on land. Over millions of years, these ancestral populations moved further and further into the water, slowly developing traits to help them swim and catch prey in aquatic environments. Today, whales have only tiny skeletal remains where their back limbs used to be, have streamlined their shape, and have developed a powerful fluke at the back of their tail to propel themselves through the water.
Influence on Physiology
Not all traits are morphological. Many traits related to metabolism, intelligence, cellular function, and many other not-clearly-visible traits are also determined genetically because they are essentially caused by the same process of protein function. Since this is determined by genetics, many physiological traits respond to the same mechanisms of natural selection imposed on morphological traits.
An interesting way to show physiological traits is to discuss how genes can be placed within bacteria, which is the way medical insulin is produced. A plasmid (a small circle of DNA that can be created artificially) with two genes is created. One gene produces a protein necessary for the bacteria to survive a particular type of antibiotic. The second gene codes for human insulin. Bacteria cells are heated up, allowing the plasmid to slip through the cellular membrane and into the cytoplasm of the cell. Thus, when the cellular machinery starts creating proteins, it also creates the proteins coded by the new plasmid. If grown on an agar plate containing the specific antibiotic, only bacteria with the plasmid will survive – allowing scientists to create entire cultures of insulin-producing bacteria!
What to Avoid
This NGSS standard also contains the following Assessment Boundary:
Assessment does not include other mechanisms of evolution, such as genetic drift, gene flow through migration, and co-evolution.
Here’s a little more specificity on what that means:
Genetic Drift:
Genetic drift is the (sometimes random) change in allele frequency over time. Genetic drift is also seen in founder populations and population bottlenecks, which can create massive changes in the allele frequency of populations. These topics are outside of the scope of this standard, but for interested students, the work of Peter and Rosemary Grant on Galapagos Finches is very interesting and displays this mechanism well.
Migration
Migration refers to the transfer of individuals from one isolated population to another. Though we typically think of species as “one population” (at least at the high school level), there are actually many different smaller populations within a species that share a gene pool. Sometimes, the allele frequencies between these two groups can vary, and migration from one group to the other can introduce variability and change the course of evolution.
Coevolution
Coevolution refers to how the evolution of one species can influence another species. This is easily seen in the “arms races” many predators and prey species have with each other. When the prey develops a defense mechanism, the predator develops an adaptation to overcome that defense and both species influence the evolution of the other. This is well-documented in bats and moths, which have a high number of defenses and adaptations that have evolved in consequence of sharing an environment with the other. This is a fun and interesting story, but outside the scope of this standard.