AP Biology 5.6 - Chromosomal Inheritance
In this final section of the AP Biology unit on Heredity, we’ll take a look at chromosomal inheritance and how it influences the heredity of genetic diseases. We’ll start reviewing chromosomal inheritance. Then, we’ll see how different genetic disorders can be caused both by mutations in specific genes and nondisjunction events that lead to genomes with too many or too few chromosomes. In this section, we’ll also review how to read a pedigree. We’ll see several common pedigree examples and learn about some different clues that can help us identify which type of inheritance pattern is at play (autosomal dominant, autosomal recessive, sex-linked, etc.). Finally, we’ll see specific examples of how nondisjunction events can cause genetic disorders in humans, and how they sometimes lead to speciation events in other populations of animals.
Video Tutorial
The following video summarizes the most important aspects of this topic!
To watch more tutorial videos like this, please click here to see our full Youtube Channel!
Resources for this Standard
For Students & Teachers
- Overview & Video Tutorial (This article)
- Quick Test Prep
- Crossword Puzzle
For Teachers Only
ENDURING UNDERSTANDING
SYI-3
Naturally occurring diversity among and between components within biological systems affects interactions with the environment.
LEARNING OBJECTIVE
SYI-3.C
Explain how chromosomal inheritance generates genetic variation in sexual reproduction.
ESSENTIAL KNOWLEDGE
SYI-3.C.1
Segregation, independent assortment of chromosomes, and fertilization result in genetic variation in populations.
SYI-3.C.2
The chromosomal basis of inheritance provides an understanding of the pattern of transmission of genes from parent to offspring.
SYI-3.C.3
Certain human genetic disorders can be attributed to the inheritance of a single affected or mutated allele or specific chromosomal changes, such as nondisjunction.
5.6 Chromosomal Inheritance Overview
Genetic heterochromia – a condition that causes a person’s eyes to be 2 different colors – is a result of mutated alleles in genes that control the distribution of melanin in the iris. With uneven distribution of this pigment molecule in each eye, each eye appears a different color.
While genetic heterochromia is a visually-striking condition, it is hardly the most severe genetic disorder that can be transmitted via chromosomes. In fact, genetic disorders of all kinds can be inherited on the chromosomes you get from your parents. Some of these disorders are caused by mutated alleles in specific genes, while others are caused by a disruption in the distribution of chromosomes during meiosis. The AP test will likely ask you about genetic disorders in one way or another. So, stick with us as we cover everything you need to know about chromosomal inheritance and genetic disorders!
Since we’ve already covered much of this information in previous videos, let’s quickly review chromosomal inheritance.
Chromosomal inheritance refers to the fact that genes, each one made of many nucleotides that carry genetic information in their sequence, are carried together on chromosomes. Each chromosome is duplicated during DNA replication, leading to two sister chromatids bound at the centromere.
In diploid organisms, each chromosome has a homolog from a different parental source that carries different alleles of the same set of genes. The process of meiosis first separates these homologous chromosomes, before separating the sister chromatids. This results in gametes that are haploid and carry only 1 copy of each chromosome. You can review this process in our video on section 5.1.
Keep in mind that different species have a different number of chromosomes, carrying a different number of genes. For example, the fruit fly has 4 chromosomes carrying 14,000 genes. By contrast, humans have 23 chromosomes carrying 22,000 genes. But, don’t think that chromosome number or number of genes is an indication of complexity. The adder’s tongue fern, for instance, has over 700 chromosomes.
In our previous video on section 5.3 we covered Mendelian genetics and showed how specific alleles can cause specific phenotypes. Unfortunately, specific alleles can also cause genetic diseases, as well. In fact, genetic disorders caused by mutated alleles often show the exact same genotypic and phenotypic ratios we would expect to see in any other genetic trait.
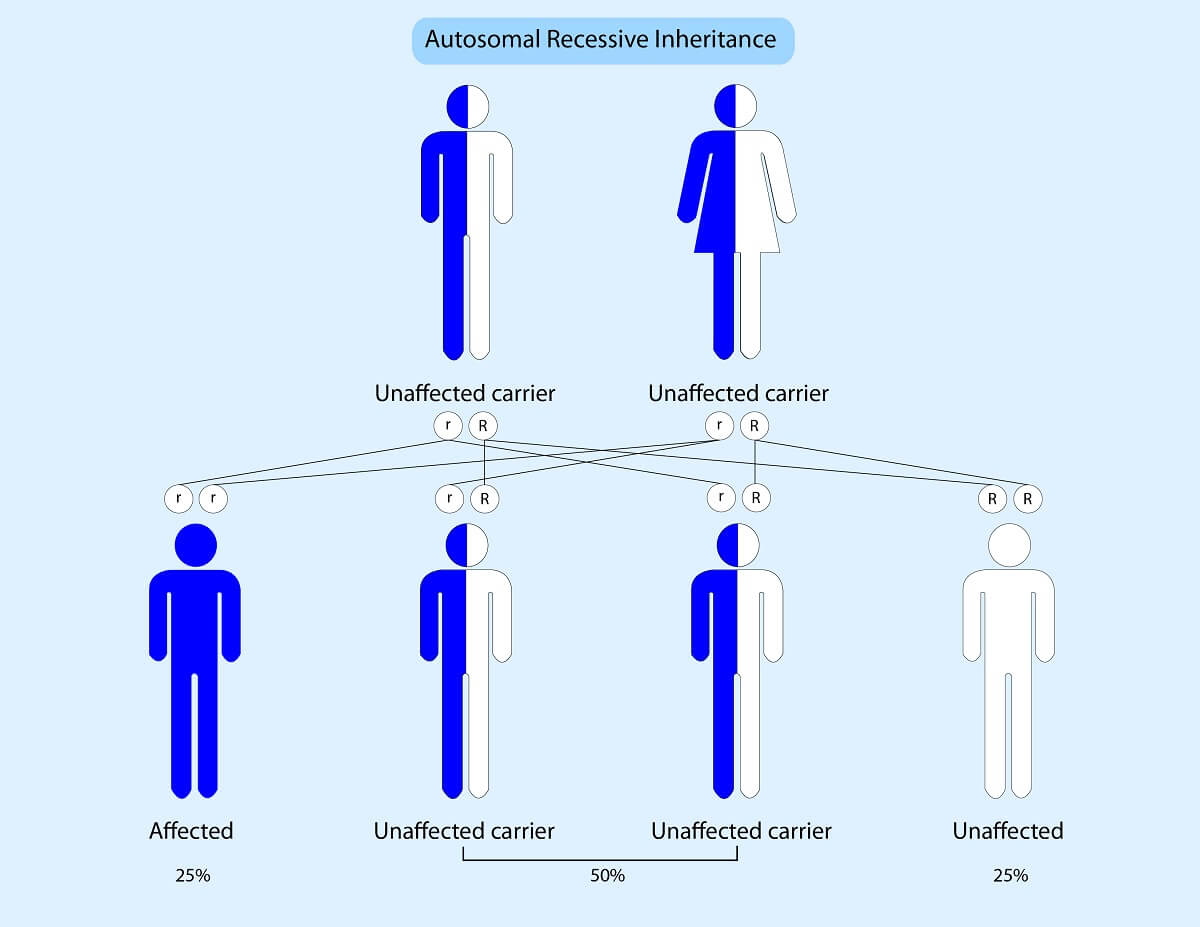
Let’s consider an autosomal recessive disorder. Just like an autosomal recessive trait, the only offspring that actually show the symptoms of an autosomal recessive disorder are those that receive two mutated, disease-causing alleles. Offspring that only receive one mutated allele are known as “carriers” – they have the ability to pass the disorder onto the next generation, but do not show symptoms themselves. So, in a cross between two carrier parents, 25% of the children will be affected by the disorder, 50% of the children will be carriers of the disorder, and only 25% of the children will be completely free of disease-causing alleles.
A good example of an autosomal recessive disorder is cystic fibrosis. The affected gene in cystic fibrosis normally codes for a protein involved with transporting chloride ions across cell membranes throughout the body. When both alleles for this gene produce dysfunctional versions of the protein, chloride ions build up in the intermembrane spaces and cause the formation of a thick mucous layer in the lungs, pancreas, and other body tissues. This leads to a myriad of symptoms, including difficulty breathing and blocked pancreatic ducts. While the disease used to be fatal within the first few months of life, modern treatments and medications have greatly extended the life of cystic fibrosis patients and future genetic therapies may be able to cure the disorder!
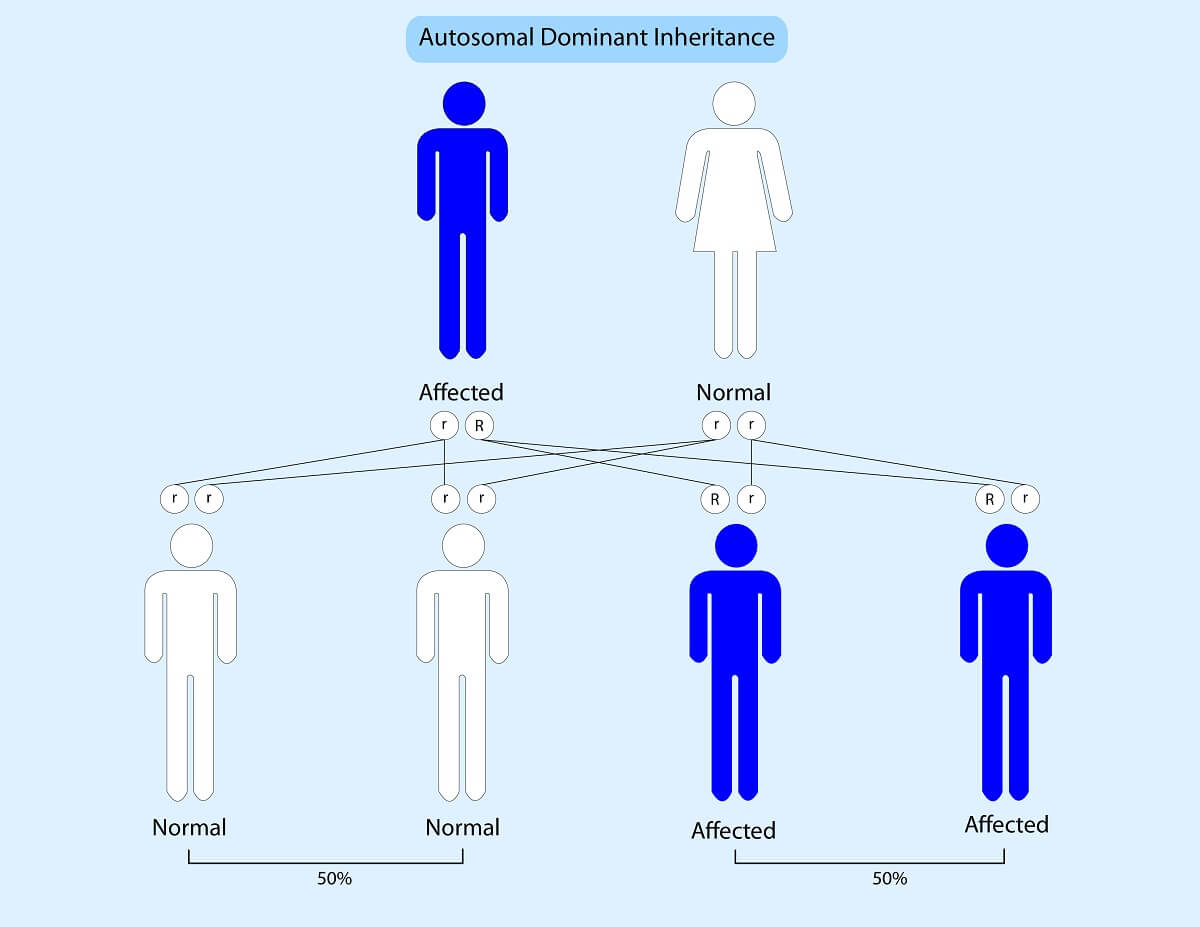
Now, let’s take a quick look at an autosomal dominant disorder like Huntington’s disease. As with normal complete dominance, even 1 dominant allele leads to disease symptoms. So, if a heterozygous affected parent and a normal, homozygous recessive individual create offspring, half of the offspring will be affected, while half of the offspring will not inherit any disease alleles. In a disease like Huntington’s disease which has a slow onset of symptoms, an affected individual may not know they have the disease until late in life. This is why genetic testing and pedigree analysis can be useful tools!
For the AP test, you will need to understand how to read a pedigree and determine the type of genetic condition that is present. Let’s start with the basics.
A square represents a male, while a circle represents a female. Horizontal lines between two individuals represent a pair of individuals making offspring, whereas vertical lines and horizontal lines above individuals represent sibling relationships. This pedigree shows the exact genotype of each individual. This is helpful in determining the exact type of inheritance pattern of a genetic disorder. Keep in mind that some pedigrees only show phenotype, which can be a little more difficult to decipher.
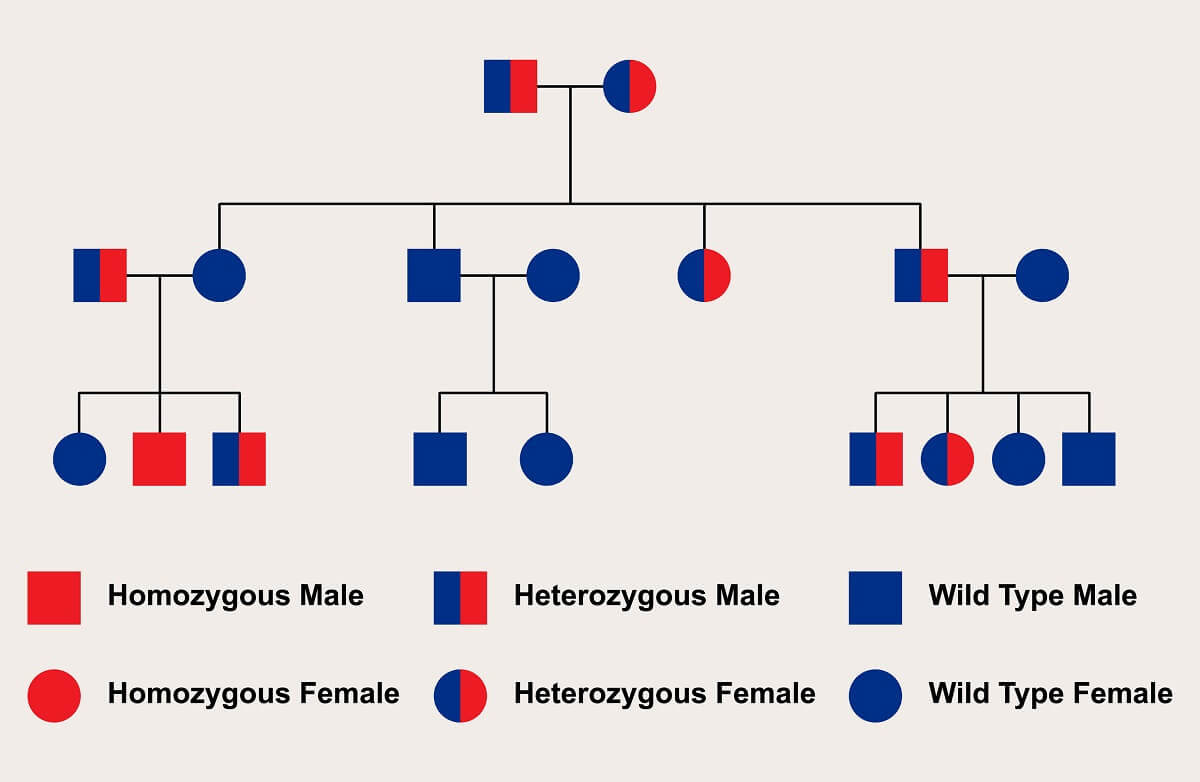
A pedigree looking at a genetic disease will also show you the affected individuals. This is how you know what genotypes cause a particular disorder. In this case, we can easily see that the only affected individual is a homozygous recessive one. Since none of the heterozygotes are affected, we know that this must be a recessive disorder. Since both males and females can be carriers, we also know that this is an autosomal disorder – if the disorder was on the Y chromosome, females could not carry it, and if the disorder was on the X chromosome, males could either be affected or not have the disease at all. Thus, we know that this particular pedigree shows an autosomal recessive disorder.
Let’s take a look at another example. This pedigree offers a few clues that can help us quickly identify the inheritance pattern of this disorder. First, we see that a cross between an affected male and a regular female produces many affected offspring. However, this pedigree gives us a second clue. A cross between two wild-type individuals produces no affected individuals.
So, let’s test a couple of different options. The affected individuals could be homozygous recessive. In this scenario, the wild-type individual would carry a dominant allele. Since affected offspring were produced, we would have to assume that the wild-type individual was heterozygous. With this arrangement of alleles, we would expect the offspring to show a 1:1 ratio of affected to wild type. The ratios are close to this, so we can’t rule it out. However, this could also be an autosomal dominant disorder, since similar ratios would be seen if the first affected male was heterozygous. Therefore, this pedigree could show either autosomal recessive or autosomal dominant patterns of inheritance. Our final clue, the fact that the trait is seen in every generation, suggests this pedigree shows a dominant trait. But, more testing or a larger pedigree would be needed to confirm this.
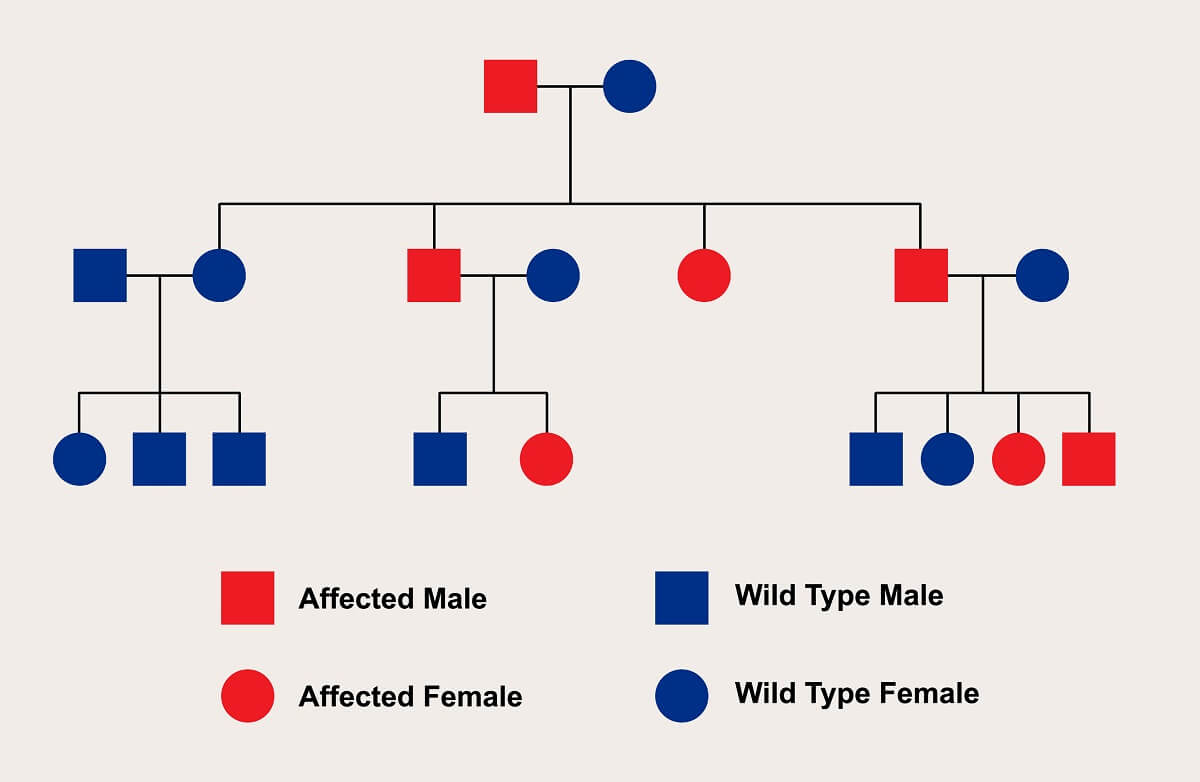
Finally, let’s see what a pedigree looks like when it shows a sex-linked pattern of inheritance. This pedigree shows a recessive condition carried on the X-chromosome. We can assume that this is an x-linked, recessive condition because males cannot be carriers, only affected or unaffected. This makes sense because each male gets only 1 X-chromosome. Further, we also see that an affected male and wild-type female produce only carrier females and healthy males. This suggests that the Y chromosome is not involved and that the females must inherit the broken allele on the X-chromosome from their father.
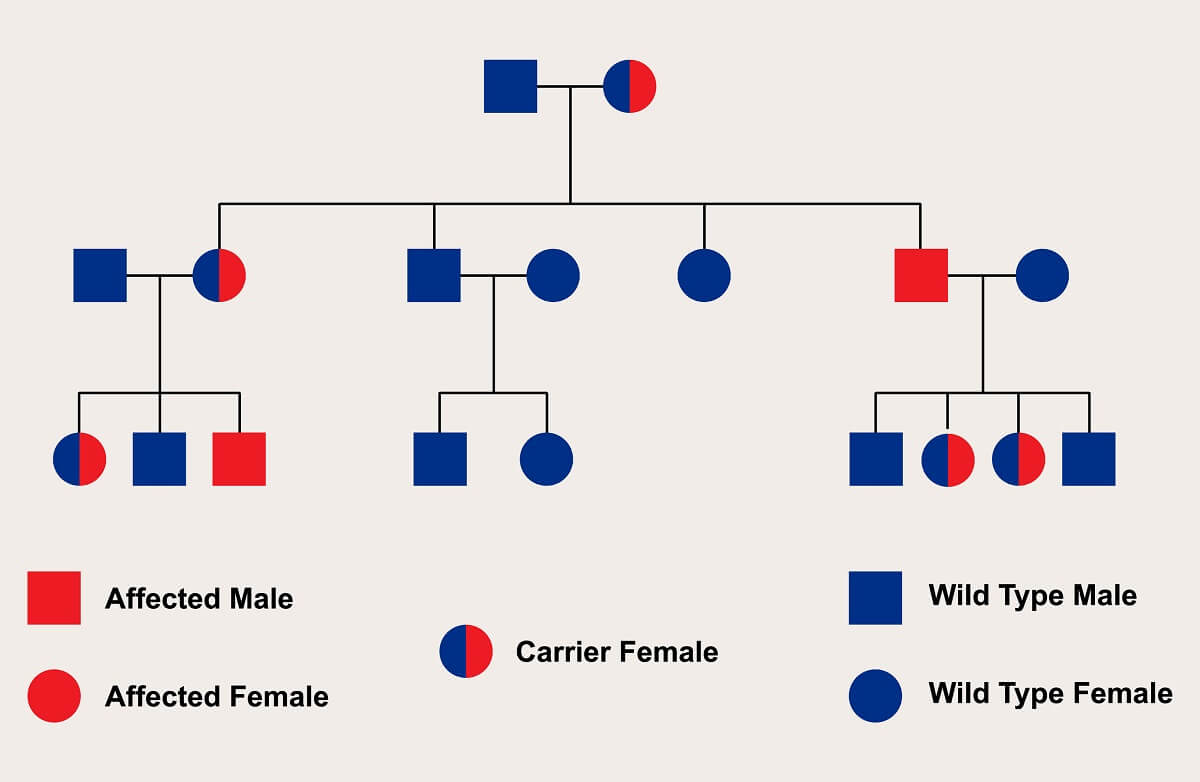
While the genetic disorders we’ve looked at previously all involved mutated alleles, other genetic disorders are not caused by mutated alleles. Rather, they are caused by abnormal meiosis events that lead to too many chromosomes in the resulting gamete. These are known as nondisjunction events, and they can happen in meiosis I or meiosis II. If a gamete with 2 copies of the same chromosome merges with a normal chromosome during fertilization, this can lead to 3 copies of the same chromosome in a single zygote – a condition known as trisomy. Trisomy 21, for example, leads to the symptoms seen in patients with Down Syndrome.
However, there are many different kinds of nondisjunction events that can lead to a wide variety of genetic disorders. For example, if a nondisjunction event leads to a gamete without a certain chromosome, this can lead to only 1 copy of the chromosome in the offspring. Turner’s syndrome is one example, where patients have only 1 X chromosome instead of 2. A similar genetic disorder is Klinefelter’s disorder, a condition caused by 2 X chromosomes and one Y chromosome.
In humans, these nondisjunction events often lead to genetic disorders. This is not true of all organisms, especially when the nondisjunction leads to organisms that have more than 2 copies of the genome. For example, nearly 80% of flowering plants evolved from a nondisjunction event that doubled the ploidy level of the resulting offspring, leading to a new species. However, many agriculture crops are produced using nondisjunction events that lead to sterile seed. Farmers sell these sterile fruits and vegetables so people can’t simply replant the seeds they buy and grow the specific varieties of fruit that farmers have carefully bred over the years for various traits.