AP Biology 3.6 - Cellular Respiration
This section of the AP Biology curriculum focuses on how cellular respiration extracts energy from the bonds of glucose (and other biological macromolecules). Unlike photosynthesis, cellular respiration is the most ubiquitous process in nature – it is present in plants, animals, fungi, and single-celled organisms. While most plants, animals, and fungi rely on aerobic respiration, most organisms also use fermentation (anaerobic respiration) as a backup for when there is no oxygen. We’ll start with an overview of cellular respiration. Then, we’ll dive into the processes of glycolysis and fermentation. Then, we’ll follow the products of glycolysis as they enter mitochondria and the Krebs cycle (citric acid cycle). Finally, we’ll see how the electron carrier molecules created throughout these initial steps of aerobic respiration go on to power an electron transport chain that generates massive amounts of ATP!
Video Tutorial
The following video summarizes the most important aspects of this topic!
To watch more tutorial videos like this, please click here to see our full Youtube Channel!
Resources for this Standard
For Students & Teachers
- Overview & Video Tutorial (This article)
- Quick Test Prep
- Crossword Puzzle
For Teachers Only
ENDURING UNDERSTANDING
ENE-1
The highly complex organization of living systems requires constant input of energy and the exchange of macromolecules.
LEARNING OBJECTIVE
ENE-1.K
Describe the processes that allow organisms to use energy stored in biological macromolecules.
ENE-1.L
Explain how cells obtain energy from biological macromolecules in order to power cellular functions.
ESSENTIAL KNOWLEDGE
ENE-1.K.1
Fermentation and cellular respiration use energy from biological macromolecules to produce ATP. Respiration and fermentation are characteristic of all forms of life.
ENE-1.K.2
Cellular respiration in eukaryotes involves a series of coordinated enzyme-catalyzed reactions that capture energy from biological macromolecules.
ENE-1.K.3
The electron transport chain transfers energy from electrons in a series of coupled reactions that establish an electrochemical gradient across membranes —
- Electron transport chain reactions occur in chloroplasts, mitochondria, and prokaryotic plasma membranes.
- In cellular respiration, electrons delivered by NADH and FADH2 are passed to a series of electron acceptors as they move toward the terminal electron acceptor, oxygen. In photosynthesis, the terminal electron acceptor is NADP+. Aerobic prokaryotes use oxygen as a terminal electron acceptor, while anaerobic prokaryotes use other molecules.
- The transfer of electrons is accompanied by the formation of a proton gradient across the inner mitochondrial membrane or the internal membrane of chloroplasts, with the membrane(s) separating a region of high proton concentration from a region of low proton concentration. In prokaryotes, the passage of electrons is accompanied by the movement of protons across the plasma membrane.
- The flow of protons back through membrane-bound ATP synthase by chemiosmosis drives the formation of ATP from ADP and inorganic phosphate. This is known as oxidative phosphorylation in cellular respiration and photophosphorylation in photosynthesis.
- In cellular respiration, decoupling oxidative phosphorylation from electron transport generates heat. This heat can be used by endothermic organisms to regulate body temperature.
ENE-1.L.1
Glycolysis is a biochemical pathway that releases energy in glucose to form ATP from ADP and inorganic phosphate, NADH from NAD+, and pyruvate.
ENE-1.L.2
Pyruvate is transported from the cytosol to the mitochondrion, where further oxidation occurs.
ENE-1.L.3
In the Krebs cycle, carbon dioxide is released from organic intermediates. ATP is synthesized from ADP and inorganic phosphate, and electrons are transferred to the coenzymes NADH and FADH2.
ENE-1.L.4
Electrons extracted in glycolysis and Krebs cycle reactions are transferred by NADH and FADH2 to the electron transport chain in the inner mitochondrial membrane.
ENE-1.L.5
When electrons are transferred between molecules in a sequence of reactions as they pass through the ETC, an electrochemical gradient of protons (hydrogen ions) across the inner mitochondrial membrane is established.
ENE-1.L.6
Fermentation allows glycolysis to proceed in the absence of oxygen and produces organic molecules, including alcohol and lactic acid, as waste products.
ENE-1.L.7
The conversion of ATP to ADP releases energy, which is used to power many metabolic processes.
EXCLUSION STATEMENT
The names of the specific electron carriers in the electron transport chain are beyond the scope of the course and the AP exam.
Specific steps, names of enzymes, and intermediates of the pathways for these processes are beyond the scope of the course and the AP exam.
Memorization of the steps in glycolysis and the Krebs cycle, and of the structures of the molecules and the names of the enzymes involved, are beyond the scope of the course and the AP Exam.
3.6 Cellular Respiration Overview
How do you make a car move? You burn gasoline in the engine, of course! The energy from the burning gasoline physically moves the engine, which sends power through the transmission to the wheels. The mitochondria within cells are essentially tiny engines that burn glucose power all the processes that cells need to survive!
In fact, mitochondria – and the process of aerobic respiration that they employ – are much more efficient than a combustion engine. Through the processes of glycolysis, the Krebs cycle, and the electron transport chain, the energy stored in the bonds of glucose is efficiently transferred to the bonds of ATP molecules. If these processes fail due to a lack of oxygen, the cell can still survive by using the process of fermentation. Since these things will certainly be on the AP test, you’d better understand them. So, stick with us and we cover everything you need to know about cellular respiration!
Cellular respiration is a metabolic process within cells that extracts energy from biological macromolecules in order to produce ATP. This ATP can then be used to power important reactions throughout the cell that help the cell maintain homeostasis, grow, and reproduce.
There are two types of cellular respiration: Anaerobic respiration and aerobic respiration. Both of these forms of cellular respiration start with the breakdown of glucose, a process known as glycolysis. Glycolysis itself releases a small amount of ATP. Then, the process of cellular respiration continues on 1 of 2 paths. If there is oxygen present, cells will carry out the process of aerobic respiration. This process sends pyruvate through the Krebs cycle, which powers the electron transport chain to produce lots and lots of ATP.
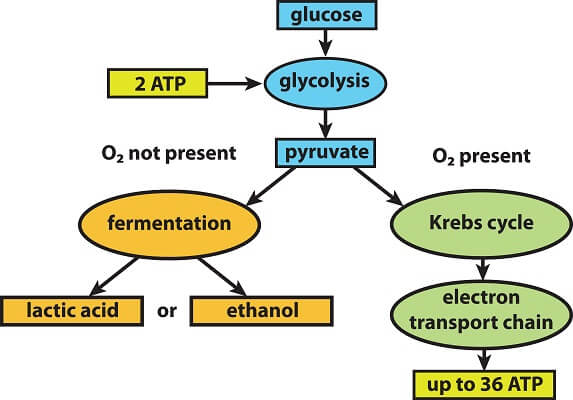
If there is no oxygen, cells carry out anaerobic respiration through the process of fermentation. Fermentation does not produce any ATP itself, though it does recycle some of the electron carrier molecules used in glycolysis. The process of glycolysis adds electrons and hydrogen atoms to these molecules, and fermentation uses these molecules to create molecules like ethanol or lactic acid. This allows the electron carrier molecules to return to the process of glycolysis, ensuring that the cell can still produce a small amount of ATP.
Humans, other animals, and plants almost exclusively use aerobic respiration. When we start to run out of oxygen (like when we are exercising or holding our breath underwater), our cells will use lactic acid fermentation to survive. But, this process can only sustain our cells for a short amount of time. Organisms like yeast can conduct alcohol fermentation and can survive for a long time while they do so. In fact, this is how alcoholic beverages are produced.
Think about this… while the complexities of cellular respiration we’re about to dive into may seem like a drag, they couldn’t be more important. Cells all over your body are constantly using cellular respiration to produce ATP to power the various things they do. The neurons in your brain are making ATP to power ion pumps that allow them to send signals. Your muscle cells are continually producing ATP to power the proteins that allow muscles to contract. Even organs you wouldn’t think about – like your kidneys – are producing a steady supply of ATP to carry out all the reactions they need to in order to keep your body in homeostasis.
As we start to go over the complex biochemistry that makes cellular respiration possible, keep in mind that you don’t have to memorize the names of specific enzymes or know all of the reactants involved. What the AP test will be testing you on is if you understand how energy is flowing through the process. With that in mind, let’s take a look at the first process in cellular respiration: glycolysis.
Glycolysis starts with an energy investment phase. During this phase, 2 ATP molecules are used to break glucose down from a 6-carbon molecule into two 3-carbon molecules of glyceraldehyde 3-phosphate. You may recognize G3P since it is also produced as a precursor to glucose via the Calvin cycle as a part of photosynthesis. (You can check out our video on section 3.6 to brush up.)
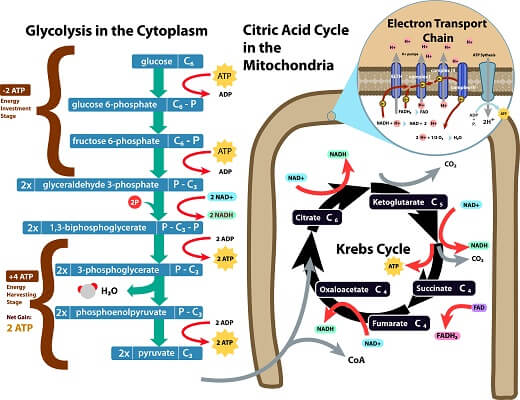
After this energy investment phase, the “payoff” or energy harvesting phase takes place. During this phase, G3P is converted into pyruvate in a process that produces 4 ATP. You should also notice that the start of this payoff stage fills 2 NAD+ electron carriers with electrons, creating 2 NADH molecules. Therefore, the total process of glycolysis produces 2 pyruvate molecules, 2 net ATP, and 2 filled electron carriers. What happens to these molecules depends on whether or not the cell has access to oxygen.
If there is no oxygen, these molecules enter the process of fermentation. Fermentation adds the electrons and hydrogen atoms from the NADH to the pyruvate molecules. This doesn’t create any more ATP, and it can leave the cell full of toxic lactic acid or ethanol if it carries on for too long. But, the process does recycle electron carriers, so the process of glycolysis can go through another cycle. This allows the cell to produce just enough ATP to survive.
However, if there is oxygen, then the products of glycolysis enter the process of aerobic respiration. Though we will look further into these processes in a second, the general overview is relatively simple. Pyruvate molecules leave the cytoplasm and enter the mitochondria of a cell. This cycle creates a small amount of ATP and produces many more filled electron carriers. These electron carriers, plus the ones created during glycolysis, then enter the electron transport chain to power the process of oxidative phosphorylation. This process produces far more ATP than any of the previous processes, allowing cells to extract the maximum amount of energy from a single molecule of glucose.
If there is oxygen present in a cell, the products of glycolysis will begin the process of aerobic respiration. This process starts with the Krebs cycle. The Krebs cycle produces carbon dioxide as it breaks pyruvate molecules down into smaller and smaller pieces. It also produces a small amount of ATP. But, most of the energy extracted in the Krebs cycle is deposited into electron carriers – including NADH and FADH2. Let’s take a quick look at the complex cycle that allows this massive transformation of energy!
The Krebs cycle is very complex, and for the purposes of the AP Biology Exam you only need to understand a few key pieces. First, at a molecular level, the cycle is a series of reactions catalyzed by specific enzymes. While some of these reactions are reversible, others can only proceed in one direction. You’ll notice that the first molecule created in the cycle is citrate, or citric acid. This is why the Krebs cycle is also known as the “citric acid cycle.”
Much like the Calvin cycle that takes place during photosynthesis, the Krebs cycle is essentially a cycle that combines carbons in energy-efficient ways. However, the goal in this cycle is to extract energy from these carbon chains, instead of store energy in the carbon chains. We can see this energy come out of the cycle at several points. Some of the energy exits the cycle as new ATP molecules. However, the large majority of the energy extracted in the Krebs cycle is placed into electron carrier molecules like FADH2 and NADH. These molecules carry the energy to the electron transport chain, which we’ll see next.
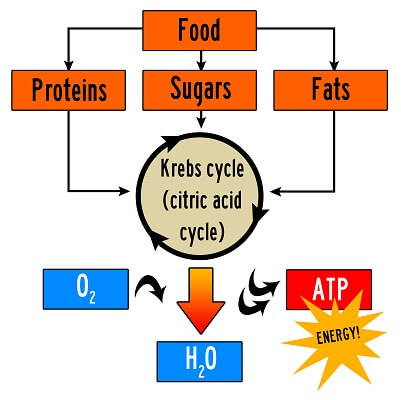
One final note on the Krebs cycle that students often misunderstand is that the Krebs cycle can process more than just glucose molecules. In fact, we typically just focus on glucose because it is the most common molecule that your body uses for energy. But, your body can also break down proteins and fat molecules into the carbon chains that they are made of. The Krebs cycle can use these molecules to power the electron transport chain, ensuring you stay alive even when there is no glucose available.
Okay, just to recap, we’re looking at the overall process of aerobic respiration, which is one of two types of cellular respiration. We’ve seen how glucose is broken down into pyruvate through the process of glycolysis. Then, this pyruvate molecule can enter the mitochondrial matrix, where it enters the Krebs cycle. Throughout this whole process, many electron carrier molecules are filled with electrons and hydrogen atoms. These electron carrier molecules make their way to the electron transport chain located on the inner mitochondrial membrane.
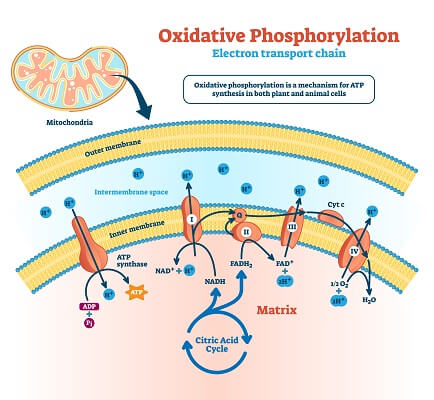
The electron transport chain starts as the electron carriers created in the Krebs cycle are separated from the hydrogen atoms and electrons that they carry. These electrons then flow through a series of integral membrane proteins on the inner mitochondrial membrane. These proteins use the energy from passing electrons to pump hydrogen ions into the intermembrane space. These hydrogen ions become concentrated in the intermembrane space, and ATP synthase can use this hydrogen ion gradient to add phosphate groups to ADP molecules to create ATP. Adding these phosphate groups to ADP molecules is known as “phosphorylation.” The entire process carried out by the electron transport chain in mitochondria is known as “oxidative” phosphorylation because oxygen is the final electron acceptor at the end of the chain.
We can compare this to the process of photophosphorylation seen in the thylakoid membrane of chloroplasts. This electron transport chain is essentially the same in that it uses the energy from electrons to create a hydrogen ion gradient that powers ATP synthase. However, this chain uses NADPH as a final electron acceptor, instead of oxygen.
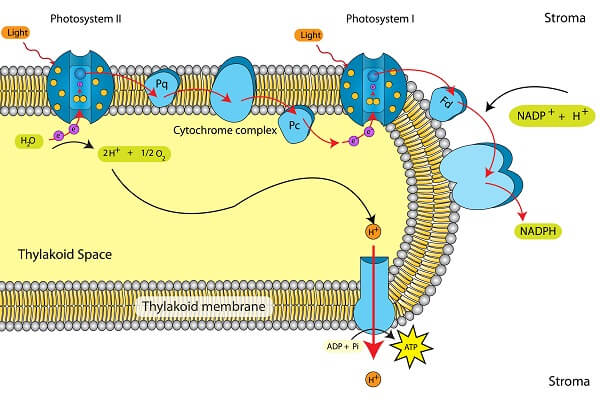
So, electron transport chains power both the storage of energy via photophosphorylation in photosynthesis and the release of that energy via oxidative phosphorylation in aerobic respiration. And that’s it! We’ve now covered the entire process of cellular respiration!